Effects of calcium propionate on the fermentation quality and aerobic stability of alfalfa silage
Article information
Abstract
Objective
To assess the potency of calcium propionate (CAP) used as silage additive, an experiment was carried out to evaluate the effect of CAP on the nitrogen transformation, fermentation quality and aerobic stability of alfalfa silages.
Methods
Alfalfa was ensiled with four levels of CAP (5, 10, 15, and 20 g/kg of fresh weight [FW]) in laboratory silos for 30 days. After opening, the silages were analyzed for the chemical and microbiological characteristics, and subjected to an aerobic stability test.
Results
The increasing proportion of CAP did not affect pH, lactic acid (LA) concentrations and yeast counts, while linearly decreased counts of enterobacteria (p = 0.029), molds (p<0.001) and clostridia (p<0.001), and concentrations of acetic acid (p<0.001), propionic acid (p<0.001), butyric acid (p<0.001), and ethanol (p = 0.007), and quadratically (p = 0.001) increased lactic acid bacteria counts. With increasing the proportion of CAP, the dry matter (DM) loss (p<0.001), free amino acid N (p<0.001), ammonia N (p = 0.004), and non-protein N (p<0.001) contents were linearly reduced, whereas DM (p = 0.048), water soluble carbohydrate (p<0.001) and peptide N (p<0.001) contents were linearly increased. The highest Flieg’s point was found in CAP10 (75.9), represented the best fermentation quality. All silages treated with CAP improved aerobic stability as indicated by increased stable hours compared with control.
Conclusion
The addition of CAP can suppress the undesirable microorganisms during ensiling and exposure to air, thereby improving the fermentation quality and aerobic stability as well as retarding the proteolysis of alfalfa silage. It is suggested that CAP used as an additive is recommended at a level of 10 g/kg FW.
INTRODUCTION
With the increasing demand of protein-rich forage, alfalfa (Medicago sativa L.) is widely cultivated and has become a major portion of the forage in diets for dairy cows in southern China, which is characterized by high temperature and rainy climate. Directly ensiling fresh alfalfa is a preferred method to conserve forage in these regions, however, fresh alfalfa silage is more prone to clostridial spoilage due to the low dry matter (DM) in addition to low sugar contents and high buffering capacity [1]. It is necessary to exploit silage additives with antimicrobial activity to ensilage high moisture alfalfa under hot and humid weather.
Attempts to develop additives with antimicrobial activity were made mainly focused on some organic acids, in particularly short-chain fatty acids [2]. Relative to other short-chain fatty acids, propionic acid (PA) used as silage additive has been considered in many studies in terms of its excellent antifungal activity and sparing impact on the activity of lactic acid bacteria (LAB) [3,4]. As an antifungal agent, PA is more effective at low pH (i.e., undissocated form) with the greatest effectiveness at or below its pKa. Inhibition of microbial growth by PA involves rapid diffusion of undissociated molecules through the plasma membrane. The dissociation of these molecules within cells liberates protons, thus acidifying the cytoplasm and preventing microbial growth [5]. Furthermore, PA could affect the nitrogen transformation and reduce the proteolysis during ensiling through acidifying silage mass or restricting activity of undesirable bacteria [6]. However, the application of PA also faces some problems due to its corrosive and hazardous nature, thus its salt derivatives have been developed and are thought to be safer and easier to handler. Calcium propionate (CAP), the salt of PA, possesses the antimicrobial effect, and offers the same efficacy as its corresponding acid after being ionized in water [7]. Therefore, the use of CAP as silage additive is assumed to be preferable compared with its acid during silage making in practice.
Evaluations of the application of PA/salt have been made mainly on high-moisture corn silages, which observed that PA/salt could suppress undesirable bacteria and improved the aerobic stability of silages [8]. However, as the effectiveness of chemical additives may vary hugely among ensilage materials, limited information is available about the recommended application rate of CAP for alfalfa silage. In addition, considering the hot and humid environmental conditions encountered during summer, it is difficult to achieve sufficient DM content in a by wilting. We hypothesized that CAP with antimicrobial attributes could be used as an additive to modulate the fermentation and inhibit the undesirable bacteria during the ensiling.
This study was aimed to evaluate the effects of varying con centrations of CAP on nitrogen compound compositions, fer-mentation quality and aerobic stability of high moisture alfalfa.
MATERIALS AND METHODS
Ensiling material and silage preparation
Alfalfa was cultivated in experimental plots at Anhui Science and Technology University (32°52′ latitude N, 117°33′ longitude E, FengYang, Anhui, China), harvested at early flowering stage and chopped into a theoretical length of 1 to 2 cm using a chopper, then thoroughly mixed by hand. The chopped alfalfa was ensiled with CAP (≥98%, Hubei Ju Sheng Technology Co., Ltd., Hubei, China) at 0, 5, 10, 15, and 20 g/kg of fresh weight (FW) (defined as control, CAP5, CAP10, CAP15, and CAP20, respectively). About 780 g alfalfa was packed into plastic laboratory silos (1-L capacity, 9.5 cm diameter×18.7 cm height, Lantian biological experimental instrument Co., Ltd., Jiangsu, China) with a density of 190.3 kg DM/m3, followed by sealing with two screw tops (internal and external). Twelve silos for each treatment were made and stored at ambient temperature (28°C to 33°C). All silos were opened after 30 days of ensiling, six silos were used for the analysis of fermentation quality and the other six silos were for aerobic stability test.
The silos used for aerobic stability test were operated as the following: alfalfa silages from each silo were emptied and placed into separate new sterile plastic larger silo (5 L capacity, 17.3 cm diameter×26.5 cm height, Lantian biological experimental instrument Co., Ltd., Jiangsu, China) without compaction. Then the larger silos were kept uncovered and stored for 7 days at ambient temperature (28°C to 33°C), which is the temperature range of summer in this region. The ambient and silage temperatures were recorded two-hourly by a glass thermometer. Aerobic stability was defined as the number of hours that the silage remained stable before its temperature increased by 2°C above ambient temperature.
Chemical and microbiological analyses
At sampling, fresh and silage samples were divided into 3 sub-samples. The first sub-sample was immediately dried in a lyophilizer to determine DM, then ground to pass a 1 mm screen in a laboratory knife mill (FW100, Taisite Instrument Co., Ltd., Tianjin, China) for subsequent analyses. The DM losses were estimated by the difference of DM contents before and after ensiling. The water soluble carbohydrate (WSC) was determined using the method of Thomas [9]. Total nitrogen (TN) was determined according to Kjeldahl procedure, Crude protein content was calculated by multiplying TN by 6.25.
Another sub-sample (35 g) was blended with 70 mL deionized water and extracted at 4°C for 24 h. The extract was filtered through four layers of cheesecloth and a filter paper (Xinhua Co., Hangzhou, China), and the pH values were measured in the filtrate immediately using a glass electrode pH meter (HANNA pH 211; Hanna Instruments Italia Srl, Villafranca Padovana, Italy). A 10-mL aliquot of 25% (w/v) trichloroacetic acid (TCA) was added to 40 mL of the filtrate. The TCA treated solution was kept at 4°C in a refrigerator overnight to precipitate the protein and then centrifuged at 18,000×g for 15 min. The supernatant was retained for the determination of free amino acid N (FAA-N), peptide-N, and ammonia N. A modified ninhydrin colorimetric assay was used for the quantification of FAA-N described by Winters et al [10]. Peptide-N was determined in the supernatant after digesting with 6 M HCl for 21 h at 105°C under an N2 atmosphere, and the amounts of peptide-N was expressed as the increase in amino acid N [11]. Ammonia-N contents were determined using the method of Shao et al [12]. Another 10-mL aliquot of TCA-treated supernatant was used for the measurement of non-protein N (NPN) by Kjeldahl procedure similar as that used in TN measurement. The organic acid and ethanol content was quantified in the filtrate using high-performance liquid chromatography (Carbomix H-NP5 column, 55°C, 2.5 mM H2SO4, 0.5 mL/min) according to the method of Yuan et al [13].
The remaining sub-sample was used for the determination of microbial populations. About 10 g of the fresh or silage sample was blended with 90 mL of sterilized saline solution (0.85% NaCl), and serially diluted from 10−2 to 10−6. The LAB was counted on de Man, Rogosa and Sharpe agar medium incubated in an anaerobic incubator at 37°C for 3 days. Yeasts and molds were enumerated on potato dextrose agar acidified with sterilized tartaric acid solution to pH 3.5 after incubation at 37°C for 3 days. Enterobacteria were counted on Violet Red Bile Agar at 37°C for 3 days. Clostridia were enumerated on tryptose sulfite cycloserine agar in an anaerobic incubator at 35°C for 24 h.
Flieg’s point calculation
Silages’ quality was assessed by the index of Flieg’s point which was calculated by means of the pH values and DM of the silages, measured at the end of ensiling with the following equation [14]:
According to that index, silage was considered to be very in ferior when it had score of <20; to be inferior with a score between 21 and 40; to be medium with a score between 41 and 60; to be good with a score between 61 and 80 and to be very good when it had score between 81 and 100.
Statistical analysis
Analyses were performed using the general linear model procedure of SAS (SAS Institute Inc., Cary, NC, USA). Data on chemical, microbiological composition and aerobic stability test were subjected to one-way analysis of variance. The polynomial orthogonal contrasts (linear and quadratic) were used to determine the response to increasing proportion of CAP. Means were compared by Tukey’s test, and differences were considered significant when p<0.05.
RESULTS
Forage characteristics
Chemical composition and microbial populations of fresh alfalfa are shown in Table 1. The alfalfa had a DM of 244 g/kg FW, and the contents of WSC and CP were 49.8 and 209 g/kg DM, respectively. Alfalfa contained 5.76 log10 cfu/g FW of epiphytic LAB, and the populations of yeast, molds, enterobacteria, and clostridia were 3.56, 4.05, 7.05, and 2.49 log10cfu/g FW, respectively.
Fermentation characteristics and microbial populations
The fermentation quality and microbial populations of alfalfa silages after 30 days of ensiling are presented in Figure 1 and Table 2. In comparison with control, addition of CAP did not significantly affect pH (Figure 1) and LA concentrations, whereas linearly decreased the acetic acid (AA) (p<0.001), PA (p<0.001), butyric acid (p<0.001), and ethanol (p = 0.007) concentrations. A significant linear decline was observed in enterobacteria (p = 0.029), molds (p<0.001), and clostridia (p<0.001) counts as increasing proportion of CAP, while not in yeast counts. There is a quadratic effect on PA (p<0.001), ethanol (p = 0.020), LAB (p = 0.001), molds (p<0.001), and clostridia (p = 0.011) as increasing proportion of CAP.
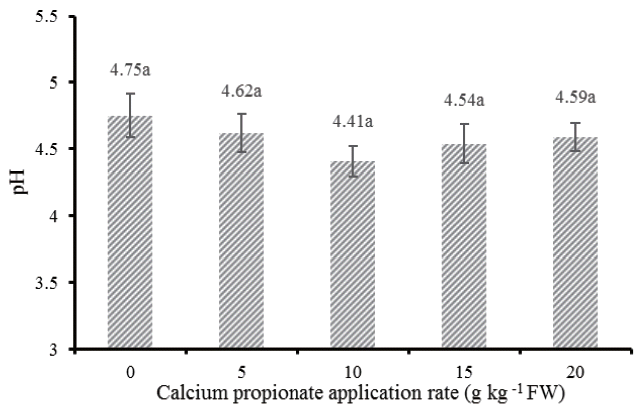
The pH of alfalfa silages treated with different levels of calcium propionate; Vertical bars are the standard errors of the means, bars with uncommon letters differ (p<0.05, n = 6).
Chemical compositions and nitrogen fractions
As presented in Table 3, the increasing proportion of CAP linearly decreased DM loss (p<0.001), FAA-N (p<0.001), ammonia N (p = 0.004), and NPN (p<0.001) contents, while linearly increased DM (p = 0.048), WSC (p<0.001), and peptide-N (p<0.001) contents. A quadratic effect on DM loss (p = 0.013) and FAA-N content (p<0.001) with increasing proportion of CAP was observed.
Flieg’ point and aerobic stability
As shown in Figure 2, the highest score was observed in CAP10 (75.9), while the lowest score was in control (60.0) that represented a medium grade.
The aerobic stability of alfalfa silages is illustra ted by the Figure 3. All silages treated with CAP improved the aerobic stability compared with control, of which CAP5 improved 10 h, while CAP10, CAP15, and CAP20 improved 36, >38, >38 h, respectively.
DISCUSSION
With the purpose of better modeling farmers’ systems of practices in southern China, where it is difficult to achieve the ideal DM by wilting a cut crop, fresh alfalfa having a DM content of 244 g/kg was ensiled directly without wilting. The DM content of a crop is crucial for the rate and extent of the resulting fermentation, and a low DM content coupled with low WSC is readily subjected to clostridial fermentation and subsequent poor acceptance of the silage by the animals [15]. In the experiment, low DM and low WSC (49.8 g/kg DM) of fresh alfalfa were limiting factors for a successful fermentation (Table 1). However, the epiphytic LAB populations were found adequate (5.76 log10 cfu/g FW), considering the recommendation of 5 log10 cfu/g FW as a minimum required for the good fermentation before ensiling [16].
After 30 days of ensiling, although no statistical difference in pH among treatments, there was an interesting tendency: the pH was first decreased and then rebounded to higher values when increasing the application rate in excess of 10 g/kg FW. The low solubility nature of this acid salt may be responsible for the diminished effectiveness on pH decline at high levels of application. In addition, McDonald et al [1] summarized the most of buffering properties of herbage can be attributed to the anions present, such as organic acid salt and orthophosphates. The higher pH in silages treated with higher levels of CAP may be also partly attributed to the increased buffering capacity, which brought by CAP per se.
The AA concentration in control was 30.2 g/kg, which is higher than that in other studies [17]. The high numbers of enterobacteria observed in the fresh alfalfa prior to ensiling caused the AA production, since enterobacteria is facultatively anaerobic and ferments sugars to acetic acid and other products before the silo becomes anaerobic [1]. The addition of CAP linearly decreased AA and ethanol concentrations, and this reduction may be attributed to their inhibition effect on undesirable microorganisms. Woolford [18] assayed the antimicrobial spectra of PA salts, and found that salts have desirable antimicrobial properties, which is superior to the corresponding free acid.
Alfalfa is difficult to ensile successfully mainly due to high buffering capacity and low WSC, while lower DM content resulted in the ensiling of alfalfa being more challenging compared with wilted alfalfa because of the increased possibility of clostridial fermentation [1]. The butyric acid concentrations in control silages were higher than the acceptable level (2.0 g/kg DM) for well-preserved silages [19], while it decreased as increasing proportion of CAP, which suggested that clostridia growth was suppressed by the antimicrobial activity of CAP. The restriction of clostridial fermentation by CAP is also typically reflected by lower ammonia N, which is another metabolic product of clostridia. The ammonia N in all CAP treated silages were decreased to a satisfactory level (<100 g/kg TN) [20], while that in control was 109 g/kg TN.
Over the process of ensiling, DM and nutrient losses seem likely to be unavoidable and irreversible, and mainly three factors may be involved in the DM consumption and dissipation of energy, including plant respiration, aerobic microorganism growth, and clostridia growth [21]. In the present study, the addition of CAP inhibited the growth of undesirable bacteria including enterobacteria, molds and clostridia, resulted in the lower DM losses. Arbabi et al [8] found that corn silages treated with CAP showed a significantly higher DM recovery than silage without additives.
The DM loss was related to the depletion of WSC. The linear increase in preservation of WSC with increasing rate of CAP might be attributed to the antimicrobial properties of CAP, which suppressed undesirable bacteria and reduced consumption of WSC. Silva et al [22] found higher WSC contents in corn silages treated with antimicrobial properties than control after 21 days of ensiling.
Degradation of herbage protein during ensiling is a compli cated biochemical process involving a series of plant and microbial enzymes. The linear increase in peptide-N suggested that CAP may depress the hydrolyzation of peptides brought by the metabolism of microorganisms. This statement is in agreement with Yuan et al [13], who reported the silages treated with CAP had higher peptide-N compared with that of control, and they ascribed it to the suppression on activity of detrimental microorganisms at later stage of ensiling.
The FAAs are one of the primary intermediate products of proteolysis by plant enzymes during the initial stage of ensiling and represent a pool of amino acids newly degraded by plant protease and amino acids rather than being metabolized by microorganisms [23]. In this experiment, CAP reduced ammonia N because of suppression of undesirable bacteria as referred above, suggesting less FAAs were degraded to ammonia. Combined with the lower FAA-N in CAP treated silages, weaker proteolysis by plant enzymes occurred during the initial stage of ensiling. It is known that proteases are deactivated at low pH, and Jones et al [24] reported that 67% of its original activities were lost after 24 h at pHs 4 and 5. It is speculated that the lower pH in CAP treated silages was responsible for the lower activity of proteases. Li et al [6] found FAA-N released from proteolysis decreased with decreasing silage pH from 6.23 to 3.40, and they ascribed it to the deactivation of plant proteases by low pH.
Silages are prone aerobic deterioration once they are exposed to air as the result of aerobic microbial activity: lactate-assimilating yeasts initialize aerobic deterioration, indicative of the degradation of lactic acid and production of excessive heat; following opportunistic bacteria (e.g., Bacilli) and molds (e.g., Aspergillus, Fusarium, and Penicillium) grow and further aggravate the spoilage in the silage mass due to the increase of silage pH resulted from degradation of lactic acid [25]. Studies showed that alfalfa silage can remain stable and does not spoil for more than one week during exposure to air [26]. However, silages deteriorated at a faster rate in our experiment compared with the results reported in alfalfa silages from other studies [26], especially in control, which had an aerobic stability of only 130 h. This poor stability was likely due to the high ambient temperature. Because environmental conditions encountered in the tropics facilitates the aerobic deterioration of silages resulting from the intensive proliferation of undesirable microorganisms. Ashbell et al [27] compared the aerobic stability of wheat and maize silages at different temperatures, and found that the silages stayed at 30°C were more prone to aerobic deterioration than those at 10°C, 20°C, or 40°C. It is deduced that the optimal temperature for growth of yeasts, which are known as the initiator of aerobic deterioration, may be about 30°C. In the experiment, the ambient temperature (28°C to 33°C) for aerobic stability test is close to 30°C, coupled with the high yeast counts at silos opening, all these together explained the fact that the alfalfa silages without CAP were more susceptible to aerobic spoilage. Addition of CAP delayed the rise in temperature of alfalfa silages: 130 h for control vs 140, 166, >168, >168 h for silage with CAP at 5, 10, 15, and 20 g/kg FW respectively. Kung et al [28] reported additive containing buffered PA substantially improved the aerobic stability of corn silages, while Kleinschmit et al [25] found that there was no effect on improving aerobic stability of corn silages treated with the same additive, and ascribed it to the lower additive applied rates, which was not sufficient to inhibit the growth of yeasts. Our data supported this fact, marginal improvement of alfalfa silages treated with low level (CAP5) was found, while silages treated with higher levels of CAP kept more aerobically stable over the periods of aerobic test.
Flieg’s point, based on the DM content and pH found in the silages, has been used for judging the quality of silages [14]. In the present study, control silage had a Flieg’s point of 60, characterized by a typical poor fermentation quality of legume; silages with 10 g/kg FW of CAP had the greatest score (75.9), represented the best silages among all treatments.
CONCLUSION
Addition of CAP can suppress the growth of undesirable microorganism during the ensiling and aerobic to air, thereby improving the fermentation quality, aerobic stability as well as retarding the proteolysis of alfalfa silages. Thus, adding CAP to alfalfa silage may be an alternative to silage additives used in southern China and the application rate of CAP is recommended at 10 g/kg FW when producing alfalfa silages.
ACKNOWLEDGMENTS
This work was partially supported by National Natural Science Foundation of China (31402135), Natural Science Foundation of Jiangsu Province of China (BK20140717), Fundamental Research Funds for the Central Universities (KJQN201555), and the National Key Basic Research Program of China (2016YFC0502005).
Notes
CONFLICT OF INTEREST
We certify that there is no conflict of interest with any financial organization regarding the material discussed in the manuscript.