In vitro Screening of Essential Oil Active Compounds for Manipulation of Rumen Fermentation and Methane Mitigation
Article information
Abstract
The objective of this study was to investigate the effects of 11 active compounds of essential oils (ACEO) on rumen fermentation characteristics and methane production. Two trials were conducted. In trial 1, ACEO (eugenol, carvacrol, citral, limonene, 1,4-cineole, p-cymene, linalool, bornyl acetate, α-pinene, and β-pinene) at a dose of 1,000 μL/L were incubated for 24 h in diluted rumen fluid with a 70:30 forage:concentrate substrate (16.2% crude protein; 36.6% neutral detergent fiber). Three fistulated Holstein cows were used as donors of rumen fluid. The reduction in methane production was observed with nine ACEO (up to 86% reduction) compared with the control (p<0.05). Among these, only limonene, 1,4-cineole, bornyl acetate, and α-pinene did not inhibit volatile fatty acid (VFA) production, and only bornyl acetate produced less methane per mol of VFA compared with the control (p<0.05). In a subsequent trial, the effects on rumen fermentation and methane production of two concentrations (500 and 2,000 μL/L) of bornyl acetate, the most promising ACEO from the first trial, were evaluated using the same in vitro incubation method that was used in the first trial. In trial 2, monensin was used as a positive control. Both doses of bornyl acetate decreased (p<0.05) methane production and did not inhibit VFA production. Positive effects of bornyl acetate on methane and VFA production were more pronounced than the effects of monensin. These results confirm the ability of bornyl acetate to decrease methane production, which may help to improve the efficiency of energy use in the rumen.
INTRODUCTION
In ruminants, methane is a natural by-product of anaerobic respiration, and its production serves as the principal electron sink in the rumen. Methane represents a significant loss of dietary energy (Beauchemin et al., 2008). The ultimate aims in manipulating ruminal fermentation are to maximize the efficiency of feed utilization and increase ruminant productivity. Therefore, considerable research effort has focused on methods to modify ruminal fermentation. Antibiotic ionophores have been very successful in reducing energy and protein losses (Nagaraja et al., 1997). However, the use of antibiotics in animal feed is facing reduced social acceptance because of the appearance of residues and resistant strains of bacteria; thus, their use has been banned in the European Union since January 2006 (Directive 1831/2003/CEE, European Commission, 2003).
The use of plant secondary metabolites as rumen modifiers seems to be a better approach since these are natural products that might be environment-friendly and have a better acceptance among consumers (Agarwal et al., 2009). Previous studies showed that some essential oils and their compounds had potential to enhance rumen fermentation (Benchaar et al., 2007; Castillejos et al., 2007). However, the effects reported in the literature are variable and contradictory. Essential oils are complex mixtures of several individual compounds, which make it difficult to elucidate the precise mechanism of action on rumen fermentation and their use as feed additives (Busquet et al., 2005a). Compared with essential oils, only a few individual active compounds of essential oils (ACEO) have been tested for their effects on rumen fermentation and methane production (Castillejos et al., 2006; Klevenhusen et al., 2012).
Therefore, the objective of the present study was to evaluate the effects of 11 ACEO (eugenol, carvacrol, citral, limonene, 1,4-cineole, p-cymene, linalool, bornyl acetate, α-pinene, and β-pinene) on rumen fermentation and methane production.
MATERIALS AND METHODS
Experimental design and treatment
In trial 1, the effects of 11 ACEO (eugenol [99% purity], carvacrol [98% purity], citral [95% purity], limonene [96% purity], 1,4-cineole [85% purity], γ-terpinene [97% purity], p-cymene [99% purity], linalool [97% purity], bornyl acetate [95% purity], α-pinene [98% purity], and β-pinene [99% purity]), which were provided by Sigma-Aldrich Chemical (Sigma-Aldrich, St. Louis, MO, USA), on rumen fermentation and methane production were evaluated using an in vitro batch fermentation system. The compounds (1,000 μL/L) were added directly to the incubation bottles, no solvent was used.
In trial 1, 108 serum bottles (12 treatments×3 cows×3 replications) were used. In trial 2, the effects of two concentrations (500 and 2,000 μL/L) of bornyl acetate, the most promising ACEO from trial 1, were evaluated using the same in vitro incubation method as in the first trial. In trial 2, monensin (10 mg/L; M5273, Sigma-Aldrich Chemical, USA) was used as a positive control. Monensin (15 mg) was dissolved in 1.5 mL of ethanol, and 0.15 mL of stock solution was added to the bottle to achieve a final concentration of 10 mg/L of culture fluid. In trial 2, 36 serum bottles (4 treatments×3 cows×3 replications) were used. In both trials, controls were included in parallel that contained neither ACEO nor monensin.
Ruminal inoculum, substrate, and in vitro incubation
Ruminal inoculum was obtained 2 h after the morning feeding from three ruminally fistulated Holstein dairy cows. In trial 1, all three cows were in lactation, whereas in trial 2, two cows were in lactation and one was in the early dry period. Cows were fed ad libitum. The total mixed ration (TMR) of lactating cows was composed (percent dry matter) of maize silage (35.5%), alfalfa silage (16%), concentrate mixture (41%), and brewers grain (7.5%). In trial 2, TMR of early dry cow was composed (percent dry matter) of maize silage (39%), alfalfa silage (18%), concentrate mixture (22%), grass hay (17%), and wheat straw (4%). The rumen content from each cow was brought separately to the laboratory in vacuum flasks, strained through one layer of cheesecloth, and used within 20 min.
The experimental substrate consisted of three feeds (alfalfa silage, maize silage, and concentrate) in a ratio of 350:350:300 mg on a dry matter basis.
In vitro fermentations were conducted in serum bottles. Briefly, 1 g of dried substrate with additives was incubated in 300-mL capacity gas-tight serum bottles containing 100 mL of phosphate-bicarbonate buffer according to McDougall (1948) with modifications (per liter of distilled water: 9.8 g NaHCO3; 7 g Na2HPO4·12 H2O; 0.6 g urea; 0.6 g KCl; 0.03 g CaCl2; 0.06 g MgSO4·7 H2O) and 50 mL rumen fluid. Fermentation bottles without additives, but containing 1 g of the substrate, were used as a control. Bottles with substrate, additives, and incubation medium were flushed with CO2 and incubated for 24 h at 39°C after sealing with a butyl rubber stopper and aluminum caps.
Sampling and analysis
After 24 h incubation, the gas in the headspace of the bottles was analyzed for methane using a gas chromatograph (GC 82F, Labio, Prague, Czech Republic) equipped with a flame ionization detector and capillary column. A sample of 0.1 mL of gas was injected using a 1 mL Sample-Lock syringe (Hamilton, Reno, NV, USA).
After opening the incubation flask, pH was measured (pH 700, Eutech Instruments, Singapore, Singapore), and 2 mL of incubation medium was collected and centrifuged for 1 min at 13,000 rpm. Supernatant (64 μL) was mixed with 736 μL of H2O, 30 μL of internal standard (2-ethylbutyric acid), and 100 μL of formic acid and then centrifuged again for 1 min at 13,000 rpm. Samples were stored at 8°C until volatile fatty acids (VFA) analysis using gas chromatography on a Labio GC 82F equipped with a flame ionization detector and capillary column, and with hydrogen as the carrier gas. Briefly, 1 μL was injected; the injector temperature was 200°C, and the inlet pressure was 50 kPa. The temperature program was 75°C at the start of the injection, increased 5°C/min until 80°C (kept for 1 min), and then increased 6°C/min until 128°C (kept for 10 min). The detector temperature was 200°C.
Chemical analysis
Samples of alfalfa and maize silage were dried in a forced air oven at 65°C. Subsequently, silages and concentrate samples were ground to pass a 1-mm sieve. Ground samples were stored for chemical analyses. The chemical composition of substrate was determined according to AOAC (2005) for crude protein (as 6.25×N), starch, and ash, and according to AOAC (1995) for ether extracts. The neutral detergent fiber (NDF) and acid detergent fiber (ADF) were measured according to the method of Mertens (2002). The ADF and NDF were assayed with a heat stable amylase and expressed exclusive of residual ash.
Statistical analysis
All statistical analyses were conducted using Statistica 9.1 (StatSoft, Tulsa, OK, USA). Data were analyzed using two-way analysis of variance using a mixed model where ACEO (treatment) was considered as the fixed factor and the cow as the random factor. The effects of ACEO on methane production, VFA production and pH were compared with those of the control using the Dunnett test (p<0.05).
RESULTS
The overall chemical composition of the experimental substrate and the chemical composition of individual feeds are presented in Table 1.
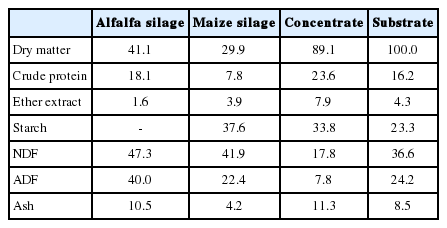
Chemical composition of alfalfa silage, maize silage, concentrate, and experimental substrate (% DM, except DM: %)
Results of the effects of ACEO (1,000 μL/L) on in vitro methane production and rumen fermentation characteristics are presented in Table 2 and 3. Among the ACEO investigated, only carvacrol treatment resulted in a pH change compared with the control (p<0.05). Nine ACEO decreased methane production compared with the control (p<0.05). At the treatment dose of 1,000 μL/L, the most pronounced inhibition of methane production was noted for carvacrol, with the magnitude of inhibition decreasing in the following order: carvacrol (−86%), citral (−44%), bornyl acetate (−38%), α-pinene (−29%), β-pinene (−28%), eugenol (−24%), linalool (−24%), limonene (−23%) and 1,4-cineole (−22%). Total VFA concentration was decreased (p<0.05) by eugenol, carvacrol, citral, p-cymene, linalool, and β-pinene. The addition of ACEO did not change (p>0.05) the molar proportion of acetate, with the exception of citral (Table 3). The addition of eugenol, citral, p-cymene, and linalool decreased (p<0.05) the molar proportion of propionate and increased (p<0.05) that of butyrate. γ-Terpinene also increased (p<0.05) the molar proportion of butyrate, but without an effect on the molar proportion of propionate. The acetate:propionate ratio increased (p<0.05) only upon the addition of eugenol, citral, and p-cymene. The effect of the cow was significant for all evaluated fermentation products, and as a random factor, contributed to total variability in the range of 18% to 73% (data not shown).

Effect of ACEO on pH, methane production and total VFA concentrations in in vitro rumen fermentation
Results of the effects of bornyl acetate (500 and 2,000 μL/L) and monensin (10 mg/L) on in vitro methane production and rumen fermentation characteristics are presented in Table 4 and 5. Neither bornyl acetate nor monensin affected pH compared with the control (p>0.05). Relative to the control, the addition of bornyl acetate at 500 and 2,000 μL/L decreased (p<0.05) methane production by 24% and 39%, respectively. Monensin decreased (p<0.05) methane production by 22%. Total VFA production was not affected (p>0.05) by bornyl acetate or monensin. Bornyl acetate (500 and 2,000 μL/L) decreased (p<0.05) the molar proportion of acetate and butyrate, and the 2,000 μL/L dose also increased the molar proportion of propionate. Monensin increased the molar proportion of acetate and decreased the molar proportion of butyrate, compared with the control. The effect of the cow was significant for all evaluated fermentation products, and as a random factor, contributed to total variability in the range of 52% to 88% (data not shown).

Effect of bornyl acetate and monensin on pH, methane production and total VFA concentrations in in vitro rumen fermentation
DISCUSSION
The selective inhibition of specific groups of microbes, such as methanogens, protozoa, and hyper-ammonia-producing bacteria, is regarded as the mode of action of essential oils and is applicable for the manipulation of rumen fermentation (Calsamiglia et al., 2007). Although essential oils are effective against both gram-positive and gram-negative bacteria, the outer membrane of gram-negative bacteria seems to provide some degree of protection (Davidson and Naidu, 2000), making them less sensitive to essential oils. Thus, shifts in bacterial composition may reduce overall bacterial fermentative activity and selectively favor less essential oil-susceptible gram-negative bacteria (Klevenhusen et al., 2012). In the rumen, gram-positive bacteria are largely acetate- and butyrate-producing bacteria, while Gram-negative bacteria are generally propionate-producing bacteria (Stewart et al., 1997).
The substrate content of crude protein and NDF was in accordance with the findings of Klevenhusen et al. (2012), who showed in meta-analysis of 20 studies that the content of crude protein and the amount of NDF of the incubated diets ranged from 139 to 189 g/kg dry matter and 160 to 429 g/kg dry matter, respectively. It appears that the effects of some metabolites of essential oils could depend on the type of diet used for in vitro incubation (Calsamiglia et al., 2007). Dietary NDF potentiated the effect of the ACEO on total VFA, whereas it counteracted the effects of the ACEO on acetate and propionate proportion and acetate:propionate ratio. Dietary crude protein potentiated the effect of ACEO on total VFA concentration, whereas it counteracted the effects of ACEO on molar proportions of propionate and butyrate and the acetate:propionate ratio (Klevenhusen et al., 2012).
The selection criteria to identify ACEO with positive effects on rumen microbial fermentation included decreased total methane production, decreased methane production per mole VFA, and simultaneously increased or no change in total VFA.
Eugenol is a phenylpropanoid and is one of the main compounds of clove (85%) and cinnamon oils (Davidson and Naidu, 2000). Eugenol has been shown to have antimicrobial activity against gram-positive and gram-negative bacteria (Dorman and Deans, 2000). Castillejos et al. (2006) reported that eugenol (500 mg/L) modified the proportions of VFA (decreased propionate) without affecting total VFA. In the present study, a twofold higher dose of eugenol also decreased the proportion of propionate, but simultaneously decreased total VFA. In contrast, in a continuous culture study, a low dose of clove oil (2.2 mg/L) decreased the molar proportion of acetate and branched-chain VFA and increased the molar proportion of propionate (Busquet et al., 2005b). These differences suggest that results are distinctly dose-dependent.
Carvacrol, a monoterpenic phenol, is one of the major components of oregano and thyme oils. Phenolic compounds such as thymol, carvacrol, and eugenol have been shown to possess high antimicrobial activity owing to the presence of a hydroxyl group in the phenolic structure. Carvacrol manifested antimicrobial activity against gram-positive and gram-negative bacteria (Dorman and Deans, 2000). Benchaar et al. (2007) observed that when used at a concentration of 400 mg/L, carvacrol did not have any effect on the total production of VFA but affected the molar proportions of VFA. In contrast, our findings showed that carvacrol (1,000 μL/L) strongly decreased total VFA and methane production but did not alter the molar proportion of VFA. This suggests that the antimicrobial activity of carvacrol may be too strong and nonspecific to improve rumen fermentation. A strong and nonspecific effect of carvacrol also was reported by Martínez-Fernández et al. (2013); in their study carvacrol (40, 80, 160, and 320 μL/L) linearly decreased total VFA and total gas production.
Citral is a lemon-scented acyclic monoterpene aldehyde that occurs naturally in essential oils of citrus fruits (e.g., up to 5% in lemon oil) and in a variety of herbs and plants such as lemongrass (70% to 80%) and eucalyptus (Jäger, 2009). Citral displayed moderate activity against gram-positive and gram-negative bacteria (Dorman and Deans, 2000). Treatment with 5, 200, and 500 mg/L mixtures of four ACEO (eugenol, carvacrol, citral, and cinnamaldehyde) with higher in two aldehyde-based compounds (citral, cinnamaldehyde) showed higher inhibitory effect on total VFA than phenolic-based mixtures, all combination also decreased methane production (Lin et al., 2013). In the present study, citral (1,000 μL/L) decreased methane production, but other effects (decreased VFA production and propionate proportion) were unfavorable. These results indicate lack of specificity of citral in methanogenesis.
Limonene is the most abundant monocyclic monoterpene in lemons, oranges, grapefruit, peppermint, spearmint, and other oils (Turner et al., 1999). Dorman and Deans (2000) demonstrated the antimicrobial activity of limonene, which was mainly against gram-negative bacteria. Castillejos et al. (2006) reported that limonene at 50 and 500 mg/L reduced total VFA concentration by 4.5% and 5.6%, respectively. In our study, the high dose of limonene (1,000 μL/L) decreased methane production and had no effect on VFA production. However, the methane production expressed relative to VFA production did not differ from that of the control (p>0.05).
1,4-Cineole, a monoterpene cyclic ether, is known to be a major flavor constituent of lime (Citrus aurantiifolia) and Eucalyptus polybractea. According to Patra and Yu (2012), eucalyptus oil (1 g/L) tended (p<0.1) to increase total VFA concentrations, decreased (p<0.01) the molar proportion of acetate and propionate, and increased the molar proportion of butyrate. Eucalyptus oil also decreased methane production. In our study, 1,4-cineole did not affect VFA and decreased methane production. Methane production expressed relative to VFA production did not differ from the control (p>0.05). These differences may be due to the synergistic effects of cineole and other ACEO in eucalyptus oil.
γ-Terpinene is a monoterpene and a major component of essential oils obtained from citrus fruits (Suzuki et al., 2004). There are no reports in the literature on the effects of γ-terpinene on rumen fermentation. Our results (Tables 2 and 3) indicate that γ-terpinene is not a potent rumen fermentation modifier.
p-Cymene is a monoterpene. In vitro studies have demonstrated that the p-cymene can be used as an antimicrobial against gram-positive and gram-negative bacteria (Custódio et al., 2011). Chaves et al. (2008) observed that p-cymene at a relatively low dose (20 mg/L) did not affect total VFA production or the proportion of VFA, but reduced methane production by 30%. In contrast, a higher dose (1,000 μL/L) in our study did not affect methane production, but decreased VFA and propionate production and increased butyrate production, implying a dependence of the outcome on the dose used. These changes in fermentation would not be nutritionally beneficial to the host animal.
Linalool is a terpene alcohol. The highest concentration of linalool is found in the essential oil of Ocimum basilicum (up to 75%) (Jäger, 2009). Linalool inhibits a wide spectrum of gram-positive and gram-negative bacteria (Dorman and Deans, 2000). Hristov et al. (2008) showed that Ocimum basilicum essential oil (10 and 100 mg/L) did not affect total VFA or pH in in vitro incubation. The higher dose of linalool (1,000 μL/L) in our study decreased the production of methane, total VFA, and propionate proportion but increased butyrate proportion. Butyrate production also was increased after inclusion of eugenol, citral, and p-cymene. Higher production of butyrate after inclusion of essential oil and ACEO has also been shown in previous studies (Busquet et al., 2005a; Castillejos et al., 2006). The bacterium Butyrivibrio fibrisolvens is a major cultured butyrate-producing bacterium in the rumen (Stewart et al., 1997). However, this bacterial species is very sensitive to essential oils (McIntosh et al., 2003). Some cryptic butyrate-producing bacteria might be less sensitive to essential oils and contribute to the increased molar proportion of butyrate in the rumen cultures. Alternatively, predominant butyrate-utilizing bacteria might have been inhibited by essential oils or other fermentation products, such as hydrogen gas (Patra and Yu, 2012).
As expected, monensin decreased the concentration of methane and the proportion of butyrate but, unexpectedly, increased the proportion of acetate. According to Nagaraja et al. (1997) monensin decreased the acetate and butyrate proportions and increased the propionate proportion. Lower methane production after monensin treatment in the present study could be due to other effects of monensin (than decreased hydrogen availability owing to decreased acetate production), such as inhibition of hydrogen production from formate or depressing of ciliate protozoa (Nagaraja et al., 1997).
Bornyl acetate is a monoterpenic ester. Valeriana officinalis root oil is rich in bornyl acetate (up to 34%) (Raal et al., 2007). García-González et al. (2008) demonstrated that Valeriana officinalis caused only negligible changes in ruminal fermentation in an in vitro screening assay. In contrast, bornyl acetate was the most promising agent in the present study. All doses of bornyl acetate (500, 1,000, and 2,000 μL/L) did not affect total VFA but methane production was reduced significantly. Oh et al. (1967) observed that bornyl acetate at 0.35 and 0.70 mL/L moderately inhibited rumen microorganisms in sheep and deer. Positive effects of bornyl acetate on rumen fermentation in trial 2 were more pronounced than those of monensin. The results observed in trial 2 on the proportions of individual VFA suggest that the main effect of bornyl acetate consists of decreasing the acetate and butyrate proportions. The effect of bornyl acetate on the proportion of propionate was observed only at the highest concentration (Table 5). Dorman and Deans (2000) demonstrated that presence of an acetate moiety in the structure increased antimicrobial activity of the parent compound; in the case of borneol, bornyl acetate increased the antimicrobial activity against the tested microorganism. The overall VFA production in control in our study differed in each trial. In trial 1, the VFA production was more than 20% higher compared with control in trial 2. The difference is probably related to the fact that in trial 2 one cow was in dry period and was fed ration with different composition (higher in roughage, lower in concentrate). As described Stewart et al. (1997) rumen bacteria vary greatly in their substrate specificity, and change in substrate may result in change of microbial community. Different microbial community composition could also influence effect of bornyl acetate on rumen fermentation, it seems that the effect of bornyl acetate (1,000 μL/L) in trial 1 was a bit stronger than the effect of higher dose (2,000 μL/L) of bornyl acetate in trial 2. Different susceptibility of various bacterial strains to ACEO was described by Dorman and Deans (2000).
Pinene is a bicyclic monoterpene. There are two structural isoforms found in nature: α- and β-pinene. As the name suggests, both isoforms are important constituents of pine resin. One of the sources containing the highest concentrations of α- and β-pinene is the essential oil of the fruit of juniper (Juniperis communis) with a total content of over 80% (Jäger, 2009). Chaves et al. (2008) showed that juniper oil (20 mg/L) decreased methane production in in vitro incubation by 49% and did not affect the total VFA production or molar proportion of individual VFA, which is consistent with our results: a high concentration of α-pinene decreased methane production and did not affect VFA production. Furthermore, α-pinene also is found in the essential oil of rosemary (Rosmarinus officinalis) (Jäger, 2009). Gunal et al. (2013) reported that rosemary essential oil (125, 250, and 500 mg/L) affected the molar proportion of VFA.
In our study, neither α-pinene nor β-pinene influenced VFA proportion, suggesting, that the different effects of a single ACEO compared with mixtures of ACEO in essential oils are probably due to synergistic effects of ACEO in essential oils. In our study we did not measure nutrient digestibility, but nutrient digestibility positively correlate with VFA production (Getachew et al., 2004). From this point of view decreased VFA production after inclusion of all tested ACEO (with exception of bornyl acetate) suggests a reduction in nutrient digestibility. Reducing in in vitro dry matter and neutral detergent fibre digestibilities after inclusion high doses of ACEO has been reported previously (Benchaar et al., 2007).
CONCLUSION
In summary, this study showed that most of the ACEO evaluated in vitro significantly decreased methane production. However, from nine ACEO, that reduced the production of methane, five also decreased production of VFA. At the dose tested, only limonene, 1,4-cineole, bornyl acetate, and α-pinene decreased methane production without adverse effects on VFA production, and only bornyl acetate reduced methane emission expressed per mol of VFA. Further research should elucidate if the reduction of methane production in bornyl acetate-treated rumen fluid also exists in vivo, and if this effect persists over a longer period.
ACKNOWLEDGMENTS
This work was supported by the Ministry of Agriculture of the Czech Republic. Project MZERO0714.
Notes
CONFLICT OF INTEREST
We certify that there is no conflict of interest with any financial organization regarding the material discussed in the manuscript.