Expression Profiles of Cellular Retinol-binding Protein, Type II (CRBP II) in Erlang Mountainous Chickens
Article information
Abstract
Cellular retinol-binding protein II (CRBP II) belongs to the family of cellular retinol-binding proteins and plays a major role in absorption, transport, and metabolism of vitamin A. In addition, because vitamin A is correlated with reproductive performance, we measured CRBP II mRNA abundance in erlang mountainous chickens by real-time PCR using the relative quantification method. The expression of CRBP II showed a tissue-specific pattern and egg production rate-dependent changes. The expression was very high (p<0.05) in jejunum and liver, intermediate in kidney, ovary, and oviduct, and lowest (p<0.05) in heart, hypothalamus, and pituitary. In the hypothalamus, oviduct, ovary, and pituitary, CRBP II mRNA abundance were correlated to egg production rate, which increased from 12 wk to 32 wk, peaked at 32 wk relative to the other time points, and then decreased from 32 wk to 45 wk. In contrast, the expression of CRBP II mRNA in heart, jejunum, kidney, and liver was not different at any of the ages evaluated in this study. These data may help to understand the genetic basis of vitamin A metabolism, and suggest that CRBP II may be a candidate gene to affect egg production traits in chickens.
INTRODUCTION
Both experimental animal models and results from clinical studies have demonstrated that vitamin A (retinol) and its active derivatives (retinoids) play a wide variety of roles in vertebrate embryonic body shaping and organogenesis, tissue homeostasis, cell proliferation, differentiation and apoptosis (Mark et al., 2009). They have also been implicated in ovarian follicular growth, oviductal and uterine environments, embryo and conceptus development, and ooctyte maturation (Schweigert and Zucker, 1988). Vitamin A-active retinoids are vital for many aspects of avian reproduction. Vitamin A deficiency and excess have profound effects on the number of eggs, egg weight, embryo survival, incubation and hatchability (Lin et al., 2002; Xiao et al., 2011). Neither retinol nor retinoids can be synthesized in vivo, thus they must be provided in the diet (Harrison and Hussain, 2001). The diet provides retinol as retinyl esters or as provitamin carotenoids, and majority of them are stored within the hepatic lipid droplets (Blaner et al., 1985).
The cellular retinol-binding proteins (CRBPs) are members of the intracellular lipid-binding protein (iLBP) multigene family. CRBPs are small molecular mass (~15 kDa) proteins that bind specifically to retinol and retinoic acid (Sundelin et al., 1985). CRBPs play important roles in intestinal vitamin A absorption and cellular retinol transport due to the extreme hydrophobicity of retinol, and also regulate the metabolism and homeostasis of retinoids through interaction with metabolic enzymes (Ong, 1987; Giguere, 1994). Currently, there are four known CRBPs, termed CRBP I, CRBP II, CRBP III, and CRBP IV. The best characterized of these are CRBP I and CRBP II.
CRBP II is an abundant cytosolic protein found in the small intestinal enterocytes of many vertebrates (Piantedosi et al., 2005). CRBP II binds specifically to retinol or retinal, and the CRBP II-retinal and CRBP II-retinol complexes serve as substrate not only for the conversion of retinal into retinol, but also for the conversion of retinol into retinyl esters (Suruga et al., 1999). Thus, the change in CRBP II mRNA levels in the small intestine or other tissues may influence the uptake and transport of vitamin A (Harrison, 2012). CRBP II-deficient mice show a decreased capacity in absorption of retinoids and reduced hepatic retinyl ester concentrations (E et al., 2002).
Because of the important role of CRBP II in the absorption and transport of retinol, this gene may be an important candidate gene for reproductive performance in chickens. In this study, we investigated the expression profile of CRBP II mRNA in various chicken tissues at different ages.
MATERIAL AND METHODS
Chickens and tissue collection
The chickens in this experiment represent 2 pure lines (SD02 and SD03) of Erlang mountainous chickens that originated from a native chicken breed in Ya’an, Sichuan province. They have strong adaptability, and delicious and nutritious meat but show relatively low reproductive performance (Yin et al., 2013). The lines SD02 and SD03 were reared at an experimental farm under the same conditions. All birds had ad libitum access to water, and feed was followed the “Erlang Mountainous Chicken Raising Standard”.
All procedures were approved by the Institutional Animal Care and Use Committee at Sichuan Agricultural University. Ten hens were randomly selected, and killed by cervical dislocation at the following ages: 12, 24, 32, and 45 wk post-hatch. Heart, hypothalamus, jejunum, kidney, liver, oviduct, ovary, and pituitary were collected for total RNA isolation. All freshly excised tissues were rinsed in ice-cold phosphate-buffered saline (PBS), and frozen within cryogenic tubes in liquid nitrogen, and stored at −80°C.
Egg laying rate
Egg production was recorded daily from 22 weeks of age to 45 weeks of age. Egg laying rate per week was calculated based on the total number of eggs and hens in a week.
RNA isolation, reverse transcription and real-time PCR
Frozen tissues were pulverized in liquid nitrogen. Total RNA was isolated from approximately 50 mg of fine powder using TRIzol reagent (Invitrogen, Carlsbad, CA, USA) according to the manufacturer’s instructions. The RNA quantity, quality and integrity was assessed with a RNA 6000 Nano chip using the Bioanalyzer 2100 (Agilent Technologies, Santa Clare, CA, USA). Reverse transcription was performed with 2 μg of total RNA and PrimeScript RT Master Mix Perfect Real Time (Takara, Dalian, China) according to the Manufacturer’s instructions.
Primers for the CRBP II gene and endogenous reference gene (β-actin) were designed by Primer Premier 5 according to the published chicken mRNA sequences (Table 1). The real time PCR reaction was performed in a volume of 15 μL containing 5.3 μL nuclease-free H2O, 7.5 μL SYBR Prime Ex Taq II (Takara, Dalian, China), 0.6 μL reverse and forward primers (10 μM), and 1 μL of cDNA with a iQ 5 Real-Time PCR Detection Systems (Bio-Rad, Hercules, CA, USA). Each reaction was carried out in triplicate, using the following cycling parameters: initial denaturation for 10 min at 95°C, followed by 35 cycles of 10 s at 95°C, 30 s at 58°C, and 45 s at 72°C, and with a terminal extension step for 10 min at 72°C.
Data analysis
The mRNA abundance was quantified by the 2−ΔΔCT method (Livak and Schmittgen, 2001). The calibrator for each gene was the average ΔCT value of 12 wk hypothalamus of SD02 served as the internal control. All data were analyzed using the GLM procedure of SPSS 18.0. The statistical model included the main effects of line, tissue, age, and 2- and 3-way interactions. Tukey’s test was employed to separate the means, and statistical significance was assigned at p<0.05.
RESULTS
The egg laying rate per week was shown in Figure 1. The egg laying rate increased from 22 wk to 32 wk, and reached 50% at 24 wk and peaked at 32 wk, then decreased from 32 wk to 45 wk.
The mRNA abundance of CRBP II was evaluated in heart, hypothalamus, jejunum, kidney, liver, oviduct, ovary, and pituitary at the four ages (12, 24, 32, and 45 wk) in two genetic lines of Erlang Mountainous chickens, SD02 and SD03. The data are summarized in Table 2, with separate rows for line, age, tissue, and interactions. The abundance of CBRP II mRNA was not influenced by genetic line in this study. There was a significant effect of tissue on CBRP II mRNA abundance (p<0.001). The mRNA expression of CBRP II was highest (p<0.05) in Jejunum as compared to all other tissues, with almost 20-fold greater expression than in heart and the two brain tissues (hypothalamus and pituitary). Expression was greater (2- to 10-fold; p<0.05) in liver than in heart, hypothalamus, kidney, oviduct, ovary, and pituitary. There was intermediate expression of CRBP II in kidney, ovary, and oviduct, and lowest (p<0.05) in heart, hypothalamus, and pituitary, as compared to all other tissues. Age also influenced mRNA expression of CRBP II, with greater mRNA abundance at 32 wk compared to 12 wk (p<0.05), and intermediate expression at 24 and 45 wk.
There was a significant interaction of age by tissue on CRBP II mRNA (p = 0.021). As shown in Figure 1, the abundance of CRBP II mRNA in hypothalamus, oviduct, ovary, and pituitary increased from 12 to 32 wk, peaked at 32 wk, and then decreased from 32 to 45 wk. However, there was no significant effect of age on the expression of CRBP II mRNA in the tissues of heart, jejunum, kidney, and liver.
DISCUSSION
In vertebrate species that ovulate a limited and species-specific number of follicles (e.g., mammals, reptiles, birds), it is the process of ovarian follicle selection that represents the rate-limiting stage in development and the availability of mature, viable oocytes for propagation of the species (Johnson and Woods, 2009). Although the right ovary and oviduct degenerate during development, hens still have high reproductive potential (Ayers et al., 2012). More than 2,500 follicles can be visualized in the ovary, and approximately 12,000 follicles are observed under the microscope, although only a few of them will mature to the point of ovulation (Yang, 2002). Generally, there are 150 to 180 eggs ovulated during the lifetime of the Erlang mountainous chicken, thus they have comparatively large space for genetic improvement. Ovulation is a complex physiological trait, which is not only influenced by hereditary factors, but also by environment, such as breeding management, nutritional levels, etc (Oguntunji and Alabi, 2010). Vitamin A has been reported to negatively affect ovulation, egg production, and egg quality in laying hens (Wilson, 1997; Moran Jr, 2007). As a vital intracellular factor in vitamin A transport, Xiao et al. (2011) reported that the polymorphism of c.349T>C in the CRBP II gene would be genetically advantageous to improve egg production traits (Xiao et al., 2011). Knowledge of the tissue expression pattern of CRBP II gene during different developmental stages provides information on how the gene is regulated.
In the current study, the CRBP II mRNA was detected in all eight tissues during the developmental stages, with greatest expression in the jejunum. The majority of dietary retinyl esters and provitamin carotenoids are absorbed in the jejunum through cellular retinol binding-proteins. As an important member of the CRBP protein family, CRBP II directs the conversion of retinol, generated from carotene cleavage, to retinol and subsequent esterification of that retinol to form the long-chain fatty acyl esters that are incorporated into the chylomicrons for export from the gut (Ong, 1993). High levels of CRBP II mRNA in the chicken small intestine is consistent with previous reports emphasizing the abundance of CRBP II in small intestine tissues of rats (Ong, 1984; Crow and Ong, 1985), humans (Ong and Page, 1987), fish (Cameron et al., 2002), and other breeds of chickens (Finlay and DeLuca, 1988; Goda and Takase, 1989). Thus, it is conceivable that the expression of CRBP II is essential for intestinal absorption of vitamin A.
Takase et al. (1995) have demonstrated that following birth, the absorptive cells in the intestine may be functionally immature and unable to convert ingested βcarotene to retinal. Thus, β-carotene is transferred to the liver in neonatal mammals and converted to retinal by the enzyme β-carotene dioxygenase in hepatocytes. Later, as the intestine matures, and can convert β-carotene to retinal, the high levels of hepatic CRBP II are unnecessary for the production of retinol, and are associated with decreased CRBP II levels in adult liver (Takase et al., 1995). In our study, we found that CRBP II mRNA was abundant in the liver of adult chickens, which differs markedly from that observed in mammals. This could be because in chickens, CRBP II directs the esterification or deesterification of retinol and the subsequent oxidation of that retinol to retinal, and then retinoic acid in the liver (Napoli, 1996), which may differ from that of mammals in that large amounts of β-carotene continue to be transported to the adult liver resulting in the need for high levels of CRBP II mRNA observed in the liver of our adult chickens. This pattern is agreement with Cameron’s report that CRBP II mRNA is highly expressed in the liver of adult zebrafish (Cameron et al., 2002). Moreover, the present finding that CRBP II is relatively strongly expressed in kidney at all four ages suggest that this gene may play an important role in the metabolism of vitamin A (retinol) in the kidney, perhaps because the kidney tubule is the main site if secondary absorption of the dietary vitamin A and provitamin A carotenoids (Liu and Li, 1999).
The expression of CRBP II in hypothalamus, oviduct, ovary, and pituitary increased from 12 wk to 32 wk, and dropped over time. This is possibly related to the egg production of Erlang mountainous chicken where the laying rate reached its peak at around 32 wk, and then declined gradually. Thus, there is more retinol required to maintain metabolic activity at the peak of egg production. The reproductive performance of chicken is mainly controlled by the hypothalamus-pituitary-ovary axis (Oakley et al., 2011), thus the change in hypothalamus, oviduct, ovary, and pituitary was expected. However, expression of CRBP II mRNA was not influenced by age in heart, jejunum, kidney, and liver, which could be due to an association with complex physiological functions of vitamin A. In addition to influencing the reproductive traits, retinol and retinoids can also trigger key steps during development, control maintenance of homeostasis, and induce or inhibit cellular proliferation, differentiation and death (Gronemeyer and Miturski, 2001).
CONCLUSION
In summary, we evaluated the ontogenic expression pattern of CRBP II mRNA in chickens, and found that the mRNA abundance was tissue-specific, and showed egg production rate-dependent changes. The results suggest that CBRP II may play an important role in egg production traits.
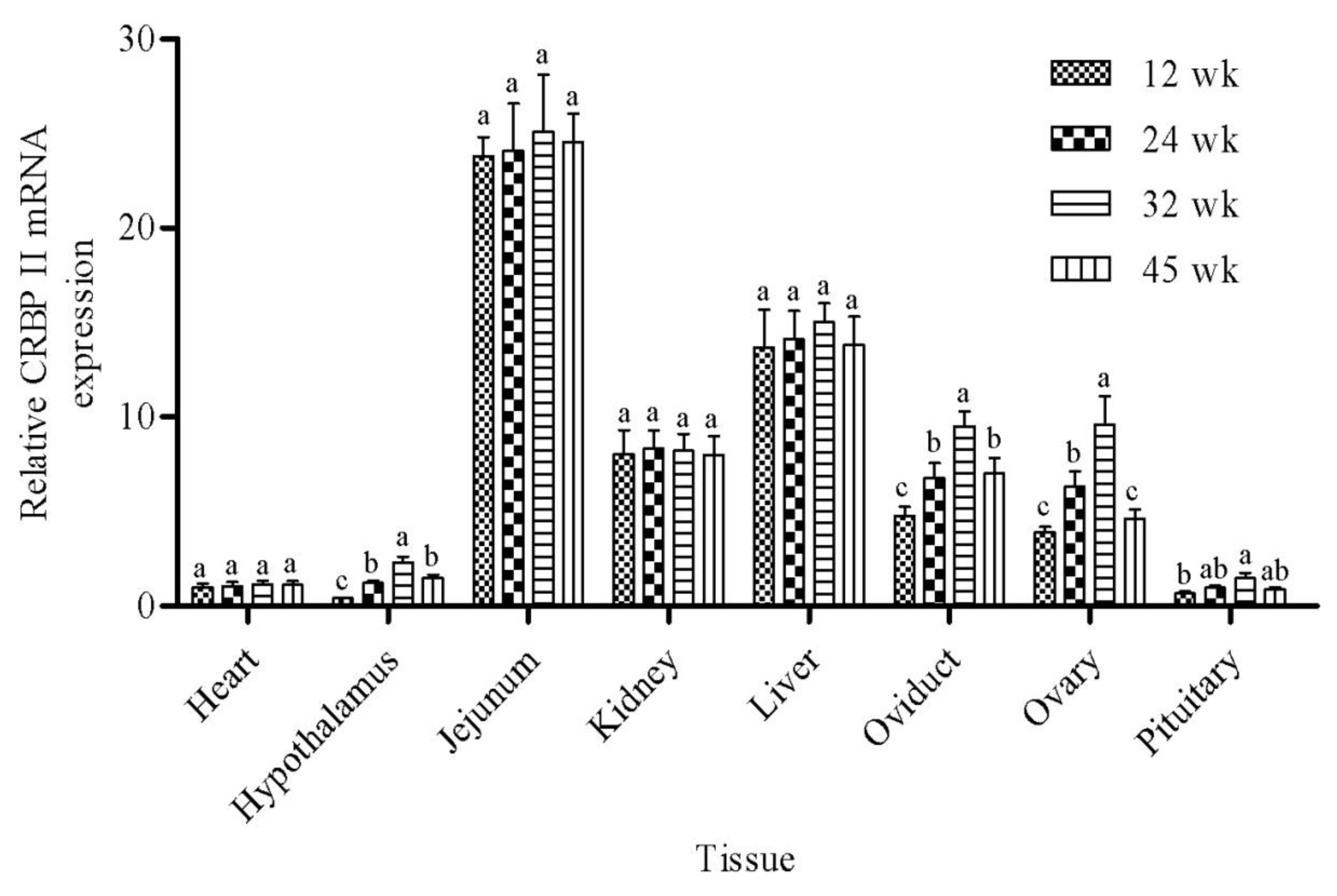
The CRBP II mRNA abundance in different tissues at 12, 24, 32, and 45 weeks (wk) of age in Erlang Mountainous chickens. Bars represent the mean±SEM. There was an interaction of age×tissue for CRBPII mRNA (p = 0.021). Different letters within the same tissue above the column indicate significant differences among time points (p<0.05).
ACKNOWLEDGEMENTS
The authors thank Jiang-Yuan Li and Yao Zhang for farming the experimental chickens. This work was supported by the China Agriculture Research System (CARS-41), the Twelfth Five Year Plan for breeding programs in Sichuan-Selective breeding of new breeds and the synthetic strains in laying hens (2011NZ0099-7), and the applied basic project of education department in Sichuan Province (10ZA114).