Effect of Grape Pomace Powder, Mangosteen Peel Powder and Monensin on Nutrient Digestibility, Rumen Fermentation, Nitrogen Balance and Microbial Protein Synthesis in Dairy Steers
Article information
Abstract
This study was designed to investigate the effect of grape pomace powder (GPP), mangosteen peel powder (MPP) and monensin on feed intake, nutrients digestibility, microorganisms, rumen fermentation characteristic, microbial protein synthesis and nitrogen balance in dairy steers. Four, rumen fistulated dairy steers with initial body weight (BW) of 220±15 kg were randomly assigned according to a 4×4 Latin square design to receive four treatments. The treatments were as follows: T1 = control, T2 = supplementation with monensin at 33 mg/kg diet, T3 = supplementation with GPP at 2% of dry matter intake, and T4 = supplementation with MPP at 30 g/kg diet. The steers were offered the concentrate diet at 0.2% BW and 3% urea treated rice straw (UTRS) was fed ad libitum. It was found that GPP supplemented group had higher UTRS intake and nutrient digestibility in terms of neutral detergent fiber and acid detergent fiber than those in control group (p<0.05). Ammonia nitrogen (NH3-N) and blood urea-nitrogen concentration were higher in monensin, GPP and MPP supplemented groups (p<0.05). Total volatile fatty acids and propionate in the GPP group were higher than those in the control group (p<0.05) while acetate concentration, and acetate to propionate ratio were decreased (p<0.01) when steers were supplemented with GPP, monensin, and MPP, respectively. Moreover, protozoal populations in GPP, MPP, and monensin supplementation were significantly lower than those in the control group (p<0.05), while cellulolytic bacterial population was significantly higher in the control group (p<0.05). Nitrogen retention, microbial crude protein and efficiency of microbial nitrogen synthesis were found significantly higher in steers that received GPP (p<0.05). Based on this study it could be concluded that the GPP has potential as an alternative feed supplement in concentrate diets which can result in improved rumen fermentation efficiency, digestibility and microbial protein synthesis in steers fed on treated rice straw.
INTRODUCTION
The amount of energy loss as CH4 within ruminant animals may account for 6% to 10% of gross energy intake, or 8% to 14% of digestible energy intake. So, in targeting methane reduction it is crucial to develop a strategy that decreases methane producing micro-biota activities and proliferation without limiting rumen function (Gemeda and Hassen, 2015). Ionophore antibiotics such as monensin are usually used to improve the efficiency of meat and milk production in ruminants. Monensin included in diets decreased CH4 production with short-term decreases in CH4 up to 30% at a dose level of 33 mg/kg diet (Guan et al., 2006). However, the use of antibiotics as feed additives has received severe criticism due to their potential health risks for consumers (Jayanegara et al., 2014). Therefore, exploration for natural and safe feed additives that mitigate CH4 emissions while increase productivity of animals simultaneously or at least without hampering the respective productivity is urgently required (Jayanegara et al., 2014).
Currently, there is increasing interest in exploiting natural products as feed additives to solve problems in animal nutrition and livestock production (Wanapat et al., 2012). Plants containing secondary metabolites, such as condensed tannins (CT) and saponins, have shown a potential to manipulate rumen fermentation by enhancing the efficiency of energy utilization in feed. One of the plants containing secondary metabolites is mangosteen peel (MP) which is a fruit by-product containing high levels of CT as well as saponins which also affects rumen fermentation (Poungchompu et al., 2009). Supplementation with MP and soap berry fruit pellet which are high in CT and saponins have been shown to alter rumen fermentation by lowering the protozoal population and CH4 production (Poungchompu et al., 2009).
Grape pomace (GP) is a high tannin feed by-product produced in large amounts in many parts of the world. The GP is usually discarded as waste and allowed to rot hence posing high environmental pollution and disposal problems in many areas including Thailand (Foiklang et al., 2015). The potential use of this waste in ruminant rations could participate in reducing the shortage of feedstuffs. In the previous study in vitro study by (Foiklang et al., 2015), it was found that supplementation of grape pomace powder (GPP) at 2% of feed increased in vitro true digestibility while reduced CH4 production. Moreover, Moate et al. (2014) has shown the supplementation 5 kg of dried grape marc to cattle could reduce gases emissions by 20% and increased milk production by 5%. However, there are limited studies on comparison of the influences of GPP and other supplements on animal performance, CH4 production and rumen fermentation in dairy steers. Therefore, the aim of this study was to investigate the effect of GPP, monensin and mangosteen peel powder (MPP) on feed intake, nutrient digestibility and rumen fermentation in dairy steers.
MATERIALS AND METHODS
Animals, diets and experimental design
Four-rumen fistulated dairy steers with initial body weight (BW) of 220±15 kg were randomly assigned according to a 4×4 latin square design (LSD). The feed ingredients and chemical composition of experimental diets and 3% urea-treated rice straw (UTRS) are shown in Table 1. All animals were kept in individual pens, mineral blocks and water were available for ad libitum consumption. The concentrate mixed diet was formulated to be at 14% crude protein (CP). The steers were offered the concentrate diet at 0.2% BW and 3% UTRS was fed ad libitum. Four dietary treatments were as follows: T1 = Control, T2 = supplementation with monensin at 33 mg/kg diet, the optimum level from the study of Guan et al. (2006), T3 = supplementation with GPP at 2% of dry matter intake, the optimum level from the previous study of Foiklang et al. (2015), and T4 = supplementation with MPP at 30 g/kg diet, the optimum level from the research of Pilajun and Wanapat. (2013). The experiment was conducted for four periods, and each period lasted 21 days. During the first 14 days, all animals were fed with their respective diets, whereas during the last 7 day, they were moved to metabolism crates for total collection of urine and feces.
Data collection, analysis and sampling procedures
At the end of each period, rumen fluid, and digesta were collected at 0, 2, 4, and 6 h-post feeding. Approximately 200 mL of rumen fluid was taken from the middle part of the rumen using a 60 mL hand syringe at 0, 2, 4, and 6 h-post feeding. Ruminal temperature and pH were measured immediately using a portable pH and temperature meter (Hanna Instruments HI 8424 microcomputer, Singapore, Singapore). Rumen fluid samples were then filtered through four layers of cheesecloth. Samples were divided into three portions, the first portion was centrifuged at 16,000×g for 15 min, and the supernatant was stored at −20°C before NH3-N analysis using the micro-Kjeldahl methods (AOAC, 1998) and volatile fatty acids (VFA) analysis using high pressure liquid chromatography (HPLC, Instruments by controller water model 600E; water model 484UV detector; column novapak C18; column size 3.9 mm×300 mm; mobile phase 10 mM H2PO4 [pH 2.5]) according to Samuel et al. (1997). The second portion was fixed with 10% formalin solution (1:9 v/v, rumen fluid: 10% formalin) for measuring microbial populations by total direct count of bacteria, protozoa and fungi using methods of Galyean (1989) with a hemocytometer (Boeco, Hamburg, Germany). The last portion was cultured using a roll-tube technique (Hungate, 1969) to identify bacterial groups (cellulolytic, proteolytic, amylolytic and total viable count bacteria).
Feces were collected and weighed during the last 7 days of each period by total collection sampling. The fecal samples were collected about 5% of total fresh weight and divided into 2 parts; first part for dry matter (DM) analysis every day and second part were kept in refrigerator and pooled by cattle at the end of each period for later chemical analysis. After being dried at 50°C for 48 h, samples were ground to pass through a 1 mm sieve in the feed mill using Cyclotech Mill, Tecator, Hoganas, Sweden for chemical analysis. The samples were chemically analyzed (AOAC, 1998) for DM, crude ash and CP. Neutral detergent fiber (NDF) and acid detergent fiber (ADF) in samples were estimated according to Van Soest et al. (1991). Contents of CT in GPP were analyzed by using the modified vanillin-HCl method, while crude saponins (CS) were measured by using methanol extraction modified by Poungchompu et al. (2009). The GP containing grape pulps and seeds were obtained from wine production factories of PB Valley Khao Yai Winery, Thailand. Total urine was collected on the same days with feces by using plastic container within drop of sulfuric acid (10%) to protect nitrogen loss. The urinary samples were collected about 10% of volume urine samples and kept in refrigerator and pooled at the end of period to be analyzed for total N (AOAC, 1998) to determine N utilization.
Blood samples (about 10 mL) were drawn from the jugular vein at the same time as rumen fluid sampling and kept into the tubes which ethylenediaminetetraacetic acid was added. Blood samples were then placed on the ice and transported to the laboratory for separating plasma from the whole blood. Samples were refrigerated for 1 h and then centrifuged at 3,500×g for 20 min (Table Top Centrifuge PLC-02, IA, USA). The plasma was removed and stored at −20°C for analysis of blood urea-nitrogen (BUN) according to Crocker (1967). The allantoin in the urine was determined by HPLC. The amount of microbial purines derivative (PD) absorption (X mmol/d) corresponding to the PD excretion (Y mmol/d) was calculated basing on the relationship derived by Chen and Gomes (1995): Y = 0.85X+(0.385W0.75). The microbial N supply (MNS) was estimated by urinary excretion of PD according to Chen and Gomes (1995): MNS (g/d) = 70X/(0.116×0.83×1,000) = 0.727X; where X is PD absorption in mmol/d, digestibility of microbial purine is 0.83, the N content of purines is 70 mg N/mmol, and the ratio of purine-N: total N in mixed rumen microbes is 11.6:100. The efficiency of microbial N synthesis (EMNS) to denote the microbial N supplied to the animal per unit of digestible organic matter apparently fermented in the rumen (DOMR) was calculated using the following formula: EMNS = MN (g/d)/DOMR (assuming that rumen digestion was 650 g/kg organic matter (OM) of digestion in total tract, DOMR = DOMI×0.65; DOMI = digestible organic matter intake).
Statistical analysis
Statistical analysis was performed using the general linear model procedure of SAS (1998). Data were analyzed using the model Yijk = μ+Mi+Aj+Pk+ɛijk, where Yijk = observation from animal j, receiving diet i, in period k; μ = the overall of mean; Mi = the mean effect of treatment (i = 1, 2, 3, 4); Aj = the effect of animal (j = 1, 2, 3, 4); Pk = the effect of period (k = 1, 2, 3, 4); and ɛijk = the residual error. Treatment means were statistically compared by Duncan’s New Multiple Range Test. Differences among means with p<0.05 were accepted as representing statistically significant differences.
RESULTS AND DISCUSSION
Chemical composition of feeds
The experimental feeds and their chemical compositions are shown in Table 1. The mixture of concentrate diet contained CP 142 g/kg DM. Concentrate ingredients were based on local resources, consisting of cassava chip, rice bran, coconut meal and palm kernel meal which had a higher quality in term of CP and also low in fiber. This concentrate was well consumed by animals during the experimental periods. GPP contained CP, CT, CS, NDF, and ADF at 128, 123, 146, 475, and 306 g/kg DM while MPP contained CP, CT, NDF, and ADF at 153, 177, 606, and 559 g/kg DM, respectively. The CP content of GPP is within the range (110 to 130 g/kg DM) reported by Yu and Ahmedna (2013). The total protein content of grape seed protein may vary significantly depending on the variety of grape, location and fertilization conditions (Yu and Ahmedna, 2013). The CT content in GPP was higher than the value reported by Moate et al. (2014). Moreover, the CT value in MPP (177 g/kg DM) was slightly higher than other values reported which ranged from 70 to 168 g/kg DM (Norrapoke et al., 2014). The inclusion of the GP into livestock rations provides an opportunity not only to use a waste by-product resourcefully, but also to induce beneficial metabolic changes in animals. The UTRS contained CP, NDF, and ADF at 55, 723, and 575 g/kg DM and was similar to that reported earlier by Anantasook et al. (2014) and Foiklang et al. (2015).
Feed intake and digestibility
Table 2 shows data on feed intake, nutrient intakes and digestibility of nutrients. Under the current study, it was found that GPP supplemented group had higher UTRS intake than those in other groups (p<0.05) in terms of percentage of BW and g/kg BW0.75. However, total dry matter intake was not significantly different among treatments (p>0.05). As observed during the experiment, both non-supplemented and supplemented animals well consumed the diet. Pilajun and Wanapat (2013) also found that adding 3% of MP (CT at 164 g/kg DM) to the buffaloes diet had no effect on feed intake. It was also found that OM, NDF, and ADF intakes were significantly higher when steers were fed with GPP (p<0.05) while CP intake was similar among treatments (p>0.05).

Effect of grape pomace powder (GPP), mangosteen peel powder (MPP) and monensin on feed intake, nutrient intakes, and digestibilities in dairy steers
In addition, nutrient digestibility in terms of NDF and ADF in GPP supplemented group were significantly higher than those in control group (p<0.05). It could be due to CT in the optimal level (20 to 40 g/kg DM) could improve nutrient digestibility (Markar, 2003) which lead to increase voluntary feed intake. However, other authors have found that feeding high levels of dietary saponins and/or tannins decreased apparent digestibility (Poungchompu et al., 2009; Bhatta et al., 2012). The negative effect of tannins on fermentation and digestion could be related to the formation of tannin–carbohydrate and tannin–protein complexes that are less degradable or toxic to rumen microbes. However, Gemeda and Hassen (2015) stated that the decreasing in fermentation and digestibility by CT levels might be due to their continuous effect during fermentation and digestibility.
Rumen fermentation parameters and blood metabolites
Measured rumen variables included temperature, pH, NH3-N, and VFA. Plasma urea N was also determined to investigate the relationship with rumen NH3-N and protein utilization. The pattern of ruminal fermentation and overall means are presented in Table 3. Ruminal temperature and ruminal pH were similar among treatments (p>0.05) while NH3-N and BUN concentration were higher in monensin, GPP and MPP supplemented group (p = 0.07). Ruminal pH and temperature were stable at pH 6.6 to 6.7 and temperature of 38.7°C to 39.1°C, and the pH was within the range considered optimal for microbial digestion of fiber and protein (Wanapat, 1990). In current study, ruminal NH3-N concentrations were 7.7 to 11.8 mg/dL and were close to those previously reported by Wanapat and Pimpa (1999) for improving rumen ecology, microbial protein synthesis, digestibility and voluntary feed intake. Increasing ruminal NH3-N concentrations also resulted in increasing concentrations of BUN. Concentrations of BUN are highly correlated to the concentration of NH3 production in the rumen and BUN concentration was influenced by treatments and the values were ranged from 7.2 to 9.2 mg/dL. Actually, monensin could reduce the NH3-N concentration in the rumen by decreasing the peptide and amino acid degradation as monensin inhibits growth of hyper-ammonia producing bacteria. However, some gram-positive bacteria may be resistant to monensin which was also observed by Krause and Russell (1996) who found no effects of monensin on Clostridium aminphilum which is a gram-positive bacterium that contributes to amino acid degradation in the rumen.
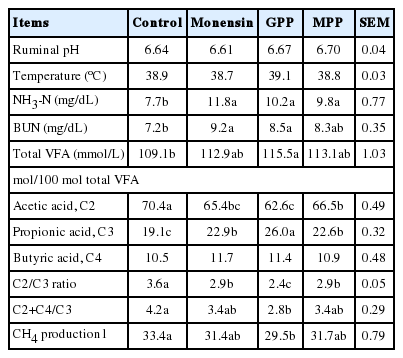
Effect of grape pomace powder (GPP), mangosteen peel powder (MPP) and monensin on rumen fermentation characteristic in dairy steers
There were differences in total VFA and VFA profiles (Table 3) when steers received monensin, GPP and MPP supplementation. Total VFA was higher in GPP supplemented group (p<0.05) while propionic acid concentrations was higher and acetic acid and acetic to propionic ratio were lower in the supplemented group than those in the control group especially in the GPP supplemented group (p<0.05) while the concentration of butyric acid was not affected by the supplements. Earlier work reported that CT and saponins had a variable effect on ruminal VFA concentration (Anantasook et al., 2014; Norrapoke et al., 2014). The effect of CT in GPP and MPP on promoting VFA concentration was very clear when animal were fed a high roughage diet. A similar shift in total VFA and propionic acid production by MP has been reported by Piajun and Wanapat (2013). The expected shift in the VFA profile from acetic to propionic was associated with shift of hydrogen from the methane pathway making it available to be used in producing propionic acid. Since fiber-degrading microorganisms are related to higher acetate production, inhibition of the cellulolytic bacteria and the anaerobic fungi species leads to lower acetate to propionate ratio (Jayanegara et al., 2014). However, the earlier work by Moate et al. (2014) stated that dried and ensiled grape marc in dairy cows diets could decrease acetic acid concentration. Calculated CH4 production was significantly lower when GPP was supplemented (p<0.05). Many previous studies reported that CT and saponins containing plants and their extracts appeared to be useful in suppressing methane release (Moate et al., 2014). It is clear that tannins had suppressed CH4 production irrespective of digestion and pattern of VFA production (Gemeda and Hassen, 2015). Monensin also is a widely used feed additive with the potential to minimize CH4 emissions from ruminants (Appuhamy et al., 2013). However, there was no reduction of CH4 production by monensin supplement in current study.
In the previous experiment, Moate et al. (2014) reported a 20% decrease in CH4 emissions and a 23% decrease in CH4 yield when either dried or ensiled grape marc was fed to dairy cows. This could be due to at least 4 substances, fat, lignin, tannins, and dl-tartaric acid, contained in GP that theoretically could be partly responsible for the observed effects. Moreover, MPP and GPP contained saponins which could decrease CH4 production.
Rumen microorganisms
Table 4 presents the rumen microorganism population data. Bacterial population and fungal zoospores were similar among treatment (p>0.05). Meanwhile, protozoal populations in GPP, MPP, and monensin supplementation were significantly lower than those in control group (p<0.05). It is possible that CT contained in GPP and MPP may play an important role in decreasing protozoal population. Consequently, rumen methanogenic bacteria could be reduced as they adhered with each other. Similarly, this data was in accordance with previous report of Norrapoke et al. (2014). The cellulolytic bacterial population was significantly lower in the supplemented groups (p<0.05) while total viable bacteria, amylolytic bacteria and proteolytic bacteria population were unchanged (p>0.05). As the data have shown that NDF digestibility of monensin and NDF/ADF digestibility of GPP supplementation were improved while cellulolytic bacteria were decreased. It could be due to another group of microbes that can play an important role in degradation of plant cell walls, namely fungi (Lee et al., 2000). Although the rumen bacteria are believed to be responsible for most of the feed digestion in the rumen because of their numerical predominance and metabolic diversity, the results obtained from Lee et al. (2000) suggested that the contribution of the fungal fraction to cell wall degradation may greatly exceed that of the bacteria. Moreover, the carboxymethyl cellulase activities of the culture supernatants for the fungi systems was also higher than that for the bacteria and protozoa monoculture systems. However, current study didn’t measured fibrolytic or cellulolytic enzymes activities. On the other hand, the number of fungal zoospores was decreased by soapberry fruit-MP pellet supplementation in dairy heifers (Poungchompu et al., 2009). The groups with GPP supplementation showed the significantly lower protozoal population than non-supplemented group. Ruminal protozoa counts were reduced through the addition of GPP and MPP in agreement with the previous work (Pilajan and Wanapat, 2013; Moate et al., 2014; Norrapoke et al., 2014). It is possible that CT contained in GPP and MPP may play an important role in decreasing protozoal population. Accordingly, protozoa provide hydrogen as a substrate for methanogenesis conducted by the methanogens. Therefore, a reduction in protozoa population (defaunation) may lead to a decrease in methanogen population and, subsequently, CH4 emission as well (Jayanegara et al., 2014). When the protozoa population is reduced in the presence of saponin-rich sources, acetate is concomitantly reduced since it is a product of protozoa metabolism from the fermentation of sugar. Further, methanogens associated with protozoa are decreased, and hence the electron transfer reaction has to search for an alternative pathway in which propionate (an alternative hydrogen sink) formation is stimulated (McAllister and Newbold, 2008).
Nitrogen utilization and purine derivatives
Effect of GPP, MPP, and monensin on nitrogen balance, purine derivatives and microbial CP supply in dairy steers are presented in Table 5. Total N intake, fecal N, urinary N and N absorption were similar among treatments (p>0.05). Meanwhile, steers received GPP and MPP showed the higher levels of N retention (32.4 and 32.2 g/d; respectively; p<0.05) and the lowest was in non-supplemented group (28.1 g/d). As previously reported by McSweeney et al. (2001) that CT improved rumen ecology especially enhancing microbial protein synthesis. Normally, when protein degradation is more rapid than synthesis, NH3 will accumulate in the rumen liquor and is absorbed into the blood carried to the liver and converted to urea and it was excreted via urine N. According to Chen and Gomes (1995), N excretion and retention could reflect on differences in N metabolism because N retention was the most important index of the protein N status in ruminant. This also could be explained by the CT containing in the GPP and MPP. Nevertheless, the positive values for the retained N with supplementation of GPP and MPP rich in CT indicate that CT supplementation met the animals’ needs for maintenance. On the other hand, Barry et al. (1986) observed that high CT content in Lotus pedunculatus increased faecal nitrogen excretion and decreased urinary nitrogen excretion, leading to higher overall nitrogen retention by sheep. Waghorn et al. (1987) suggested that increased nitrogen retention with Lotus corniculatus appeared to be due to improved utilization of absorbed nitrogen. Indeed, it has been suggested that CT might increase the efficiency of urea recycled to the rumen because CT reduce the rate of protein degradation and deamination in the rumen and therefore lower ruminal NH3. The results revealed that allantoin excretion and absorption were higher in the group of steers fed with GPP and MPP (p<0.05). Furthermore, microbial nitrogen supply and microbial CP were significantly improved (p<0.05) when steers were fed with GPP while efficiency of microbial nitrogen synthesis was significantiy higher in the GPP and monensin supplemented group (p<0.05). Urinary excretion of PD is considered to be an indicator of microbial production in the rumen.
CONCLUSION
Based on this study it could be concluded that GPP has potential as an alternative feed supplement in the concentrate diet that can result in improved rumen fermentation efficiency, digestibility, propionic acid concentration and microbial protein synthesis. In addition, GPP could reduce acetic to propionic ratio and protozoal population. Therefore, supplementation of GPP in ruminant feeding is recommended. Interestingly, GPP showed comparable results as using monensin or MPP.
ACKNOWLEDGMENTS
The authors would like to express their most sincere thanks to the Tropical Feed Resources Research and Development Center (TROFREC), Thailand Research Fund (TRF) through the Royal Golden Jubilee Ph.D. Program, Department of Animal Science, Faculty of Agriculture, Khon Kaen University and Agricultural Development Research Center of Northeast Thailand and PB Valley Khao Yai Winery, Thailand for their kind financial support and experimental facilitie.
Notes
CONFLICT OF INTEREST
We certify that there is no conflict of interest with any financial organization regarding the material discussed in the manuscript.