The Regulation of Chemerin and CMKLR1 Genes Expression by TNF-α, Adiponectin, and Chemerin Analog in Bovine Differentiated Adipocytes
Article information
Abstract
Adipokines, adipocyte-derived protein, have important roles in various kinds of physiology including energy homeostasis. Chemerin, one of adipocyte-derived adipokines, is highly expressed in differentiated adipocytes and is known to induce macrophage chemotaxis and glucose intolerance. The objective of the present study was to investigate the changes of chemerin and the chemokine-like-receptor 1 (CMKLR1) gene expression levels during differentiation of the bovine adipocyte and in differentiated adipocytes treated with tumor necrosis factor-α (TNF-α), adiponectin, leptin, and chemerin (peptide analog). The expression levels of the chemerin gene increased at d 6 and 12 of the differentiation period accompanied by increased cytoplasm lipid droplets. From d 6 onward, peroxisome proliferator-activated receptor-γ2 (PPAR-γ2) gene expression levels were significantly higher than that of d 0 and 3. In contrast, CMKLR1 expression levels decreased at the end of the differentiation period. In fully differentiated adipocytes (i.e. at d 12), the treatment of TNF-α and adiponectin upregulated both chemerin and CMKLR1 gene expression levels, although leptin did not show such effects. Moreover, chemerin analog treatment was shown to upregulate chemerin gene expression levels regardless of doses. These results suggest that the expression of chemerin in bovine adipocyte might be regulated by chemerin itself and other adipokines, which indicates its possible role in modulating the adipokine secretions in adipose tissues.
INTRODUCTION
In ruminants, adipose tissues are the major lipogenic and lipid-storing organ that regulate energy homeostasis, meat quality and lactation (Laliotis et al., 2010). The discovery of leptin secretion by adipocytes provided another aspect of the adipose tissue not only as lipid storage, but also as an endocrine organ (Friedman and Ramirez, 1994). After leptin, there were other adipokines discovered that were secreted from the adipocyte, namely adiponectin, resistin and tumor necrosis factor-α (TNF-α), etc. These adipokines are known to have various roles in physiological functions such as energy metabolism, inflammatory responses and in the vascular system. In addition to those adipokines, we previously identified another adipokine named chemerin, which is the ligand of orphan G protein-coupled receptor “CMKLR1 (chemokine-like-receptor 1)” with chemotactic properties (Wittamer et al., 2003; Roh et al., 2007). Recent studies suggest that chemerin may regulate insulin sensitivity and adipogenesis and have a potential role in the development of metabolic syndromes, such as obesity, diabetes and fatty liver disease (Kukla et al., 2010; Lehrke et al., 2009; Mussig et al., 2009). In addition, the detailed molecular mechanism of the regulation of chemerin expression has been reported (Bauer et al., 2011; Kralisch et al., 2009; Muruganandan et al., 2011). In our previous studies, chemerin was up-regulated during bovine adipocyte differentiation and chemerin analog regulated insulin secretion related to glucose metabolism and the release of triglycerides in sheep in vivo (Song et al., 2010; Suzuki et al., 2012). However, the factors regulating chemerin gene expression have not been well studied in bovine adipocytes. Therefore, this study was aimed to investigate the changes in chemerin and its receptor CMKLR1 gene expression during bovine adipocyte differentiation. We also addressed the regulatory effect of TNF-α, adiponectin, leptin and chemerin analog instead of recombinant full-length chemerin on chemerin and CMKLR1 gene expression in bovine differentiated adipocytes.
MATERIALS AND METHODS
Isolation of bovine stromal-vascular (S-V) fraction cells including preadipocytes, and induction of adipocyte differentiation
The S-V fraction cells, which have an abundant population of preadipocytes, were isolated from the subcutaneous adipose tissues of male Japanese Black cattle and then differentiated to adipocytes as previously reported by Song et al. (2010) with a modification. Briefly, confluent S-V fraction cells were incubated in DMEM/HAMF12 supplemented with 10% FBS, 1% antibiotic mix solution, octanoic acid (5.0×10−3 M), sodium acetate (10−2 M), lipid mixture (100-fold dilution), T3 (2.0×10−9 M), dexamethasone (10−8 M), insulin (10−7 M) and troglitazone (10−8 M) for additional 12 d. This differentiation medium was changed every 48 h during the adipocyte differentiation.
Oil Red O staining
Cultured adipocytes were stained with Oil Red O to observe the amount of lipid droplets in the cytoplasms. The procedure of Oil Red O staining was described previously (Song et al, 2010).
TNF-α, adiponectin, leptin and chemerin analog treatment in differentiated adipocytes
After differentiation for 12 d, adipocytes were incubated in DMEM/HAMF12 medium containing 10% FBS and 1% antibiotic mix solution for 24 h to remove the stimulus by differentiation-inducing reagents. The culture medium was then refreshed with DMEM/HAMF12 medium added to either with TNF-α (10 ng/ml), adiponectin (10 (μg/ml), leptin (10 ng/ml) or chemerin analog (10−9, 10−8 or 10−7 M). After the adipokine treatments, the adipocytes were incubated for 24 h before proceeding to RNA extraction. The chemerin analog (NH2-yFLPsQFa(Tic)S-COOH) was prepared according to the previous report by Shimamura et al. (2009).
Total RNA extraction and real time PCR
Total RNA was extracted from cultured cells using RNAiso Plus (Takara Bio, Inc., Otsu, Japan). The quality and quantity of extracted total RNA were determined with a NanoDrop ND-1000 spectrophotometer (NanoDrop Technologies, DE, USA) and agarose gel electrophoresis. Total RNA (250 ng) was reverse-transcribed to cDNA using PrimeScript RT reagent (Takara Bio, Inc., Otsu, Japan). The sequence and amplicon sizes of the primers are shown in Table 1 with 18S rRNA as the housekeeping gene. The reactions were performed in a 10 μl of final volume containing 2×SYBR Premix Ex Taq II (Takara Bio, Inc., Otsu, Japan), 0.25 mM of each primer, and 2 μl from the RT product. Real-time PCR was carried out in DNA Engine Opticon 2 Continuous Fluorescence Detector (MJ Research, Ramsey, MN, USA) with initial denaturation at 95°C for 30 s and 45 cycles of denaturation at 95°C for 5 s, annealing at 61°C for 20 s, and extension at 72°C for 15 s. Post-PCR melting curves were observed to confirm the specificity of single-target amplification, and the mRNA expression levels of the genes of interest were normalized with the housekeeping gene.
Statistical analysis
The data are expressed as the percentage of expression levels at d 0 or that of control values (mean±SEM) and represent data collected form at least three experiments. The data from d 0 to 12 of bovine adipocyte differentiation were statistically analyzed using one-way analysis of variance (ANOVA) followed by Tukey’s test. The data between control and adipokines treatment group were compared by Student’s t-test. Differences in mean values between groups were considered to be significant at p<0.05.
RESULTS
Gene expression levels of chemerin and CMKLR1 during bovine adipocyte differentiation
Oil Red O staining was performed to observe the lipid deposition in preadipocytes on d 0 and differentiated adipocytes on d 3, 6, 9, and 12. As shown in Figure 1, the adipocyte differentiation was accompanied by an increased amount of lipid droplets. In Figure 1B, the level of gene expression of PPAR-γ2 as differentiation-marker gene was upregulated almost 20 fold higher from d 6 to d 12 than that at d 0. On d 6 and 12, the gene expression levels of chemerin also increased significantly although with a slight fall at d 9. In contrast, CMKLR1 mRNA expression was decreasing on d 12.
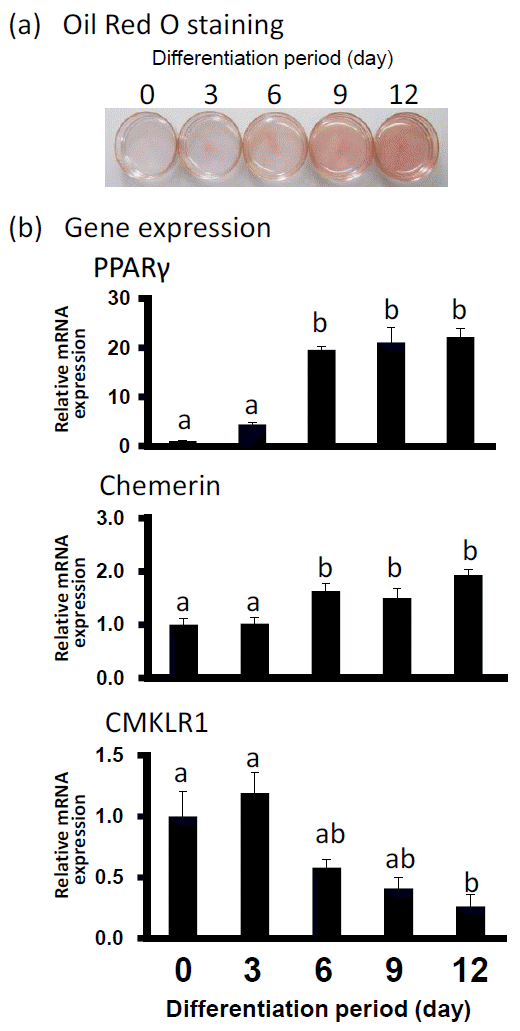
Lipid accumulation and gene expression levels of PPAR-γ2, chemerin and CMKLR1 mRNA during bovine adipocyte differentiation. (a) Oil Red O staining of bovine differentiated adipocytes at indicated days of differentiation. (b) Gene expression level of PPAR-γ2, chemerin and CMKLR1 when Oil Red O staining was performed. The data for expression analysis are shown as means±SEM and collected from at least three experiments. The data were normalized with 18s rRNA and expressed in relative to the expression levels of day 0. a,b Values marked with different letters within each gene differ significantly (p<0.05).
Effects of TNF-α, adiponectin, and leptin on gene expression levels of chemerin and CMKLR1
We investigated the regulatory effect of TNF-α, adiponectin, and leptin on chemerin and CMKLR1 mRNA expression in differentiated adipocytes. As shown in Figure 2a and 2b, TNF-α and adiponectin treatments significantly elevated the gene expression levels of both chemerin and CMKLR1 in differentiated adipocytes. However, treatment of leptin did not seem to alter the gene expression levels of chemerin and CMKLR1 (Figure 2c).

Gene expression levels of chemerin and CMKLR1 mRNA in bovine differentiated adipocytes treated with TNF-α (a), adiponectin (b) and leptin (c). The data are shown as means±SEM and represent data collected from at least three experiments. The data were normalized with 18s rRNA and shown as means±SEM in relative to the levels of control cells (* p<0.05 vs control).
Effect of chemerin analog on gene expression levels of chemerin and CMKLR1
To investigate the effect of chemerin analog on chemerin and CMKLR1 gene expression levels, differentiated bovine adipocytes were treated with chemerin analog at various doses. As shown in Figure 3, treatment of chemerin analog at 10−9, 10−8 and 10−7 M significantly upregulated the mRNA expression of chemerin but not its receptor. However, this stimulatory effect of chemerin analog in chemerin gene expressions was not shown in a dose-dependent manner.

Gene expression levels of chemerin and CMKLR1 in bovine differentiated adipocytes treated with chemerin analog (10−9, 10−8 and 10−7 M). The data are shown as means±SEM and represent data collected from at least three experiments. The data were normalized with 18s rRNA and shown as means±SEM in relative to the levels of control cells (* p<0.05 vs control).
DISCUSSION
In the present study, we have shown that the gene expression levels of chemerin and CMKLR1 in bovine adipocytes were altered during adipocyte differentiation and regulated by TNF-α, adiponectin, and chemerin analog. Our results also demonstrated that the increasing level of chemerin gene expression was accompanied with the increased amount of lipid droplets and the increased expression of PPAR-γ2. Adipocyte differentiation is known to be driven by adipogenic genes, e.g. PPAR-γ2, CCAATT enhancer binding protein (C/EBP), and sterol regulatory element-binding protein 1 (SREBP1), which serve as transcriptional factors for their downstream genes involved in adipogenesis (Fajas et al., 1998; Lefterova and Lazar, 2009; White and Stephens, 2010). It has been reported that chemerin has PPAR-γ response element (PPRE) in its promoter region and thus its gene expression is controlled by PPAR-γ (Muruganandan et al., 2010). This report is in agreement with ours where there was a simultaneous increase of PPAR-γ2 and chemerin during the adipocyte differentiation. In addition, SREBP2, a transcription factor that control sterol synthesis and is induced in adipocytes during obesity (Le Lay et al., 2004), binds to sterol regulatory element (SRE) in the promoter of chemerin gene and upregulates its expression (Bauer et al., 2011). Thus, the stimulatory effect of adipocyte differentiation on chemerin gene expression can be explained by the increased PPAR-γ2 gene expression levels and also probably by SREBP2 regulation.
As for CMKLR1, our results suggested that the expression level of CMKLR1 increased in the early stage of differentiation, but decreased later. Some studies have previously analyzed the expression of CMKLR1 during adipocyte differentiation and the results are divided into two opinions: one is the gradual upregulation of CMKLR1 gene expression levels during adipocyte differentiation (Goralski et al., 2007; Roh et al., 2007) and the other one is the downregulation of CMKLR1 gene expression at the end of differentiation, which is in agreement with our result (Bozaoglu et al., 2007; Sell et al., 2009). Given the lowered gene expression level of CMKLR1 yet with the increase of chemerin expression levels as the differentiation progressed, we speculate that chemerin might direct its signal not to adipocyte as main target tissue, but rather stimulate macrophage infiltration causing inflammation in adipose tissue. This might explain for the possible chemerin role in altered metabolism of adipose tissue.
Large numbers of studies on TNF-α have indicated its physiological effects, which related to the induction of lipolysis, systemic inflammation in adipose tissue, and subsequently insulin resistance (Guilherme et al., 2008; Suganami and Ogawa, 2010). Also, adiponectin is currently considered to be a beneficial adipokine that ameliorates systemic inflammation in adipose tissues, improves insulin sensitivity and induces fatty acid oxidation (Combs et al., 2004; Yamauchi et al., 2002). In the present study, the treatments with TNF-α or adiponectin upregulated the gene expression levels of chemerin and CMKLR1 in differentiated adipocytes. This upregulation might result in a suppressive effect on fat accumulation in adipose tissue, suggesting that chemerin is one of the adipokines which is involved in changing the adipose tissue metabolic state into an anti-lipogenic process and inducing lipolysis.
In previous studies, the physiological effects of chemerin have been demonstrated to increase lipolysis and the downregulation of PPARγ gene expression (Roh et al., 2006), which also stimulates chemotaxis of macrophages and dendritic cells (Wittamer et al., 2003). Our results showed that the chemerin analog upregulated the gene expression of chemerin. This positive feedback pathway provides further information in the regulatory mechanism of chemerin expression as an adipokine modulating inflammation and metabolism of adipose tissue in differentiated adipocytes. However, the absence of change in CMKLR1 receptor gene expression level by the chemerin analog indicates that chemerin might not directly affect the gene expression of its receptor in bovine adipocytes.
In conclusion, the upregulation of chemerin and downregulation of CMKLR1 gene expression levels during adipocyte differentiation suggests their roles in the development and lipid metabolism of adipose tissue. Furthermore, the up-regulations of chemerin and CMKLR1 gene expression levels by TNF-α and adiponectin indicate the role of chemerin in inflammation and lipolysis in adipose tissues. Also, there is a positive feedback pathway in chemerin gene expression. These present results may offer new information to clarify the role of chemerin in modulating the adipogenesis and fat accumulation in ruminant adipocytes and adipose tissues.
ACKNOWLEDGEMENTS
This work was supported by the National Research Foundation of Korea Grant funded by the Korean Government (KRF-351-2009-1-F0016).