Effects of Feeding Methylthio Butyric Acid Isopropyl Ester on Postpartum Performance and Metabolism in Dairy Cows
Article information
Abstract
The present experiment aimed to evaluate the effect of HMBi on the production performance and metabolism in dairy cows. Thirty multiparous Holstein dairy cows under similar conditions were randomly assigned to three dietary treatments; i) Control, a basal diet; ii) T1, a basal diet plus HMBi (0 g prepartum and 18 g postpartum); and iii) T2, a basal diet plus HMBi (10 g prepartum and 18 g postpartum). Treatments were initiated 21 d before expected calving and continued through 91 d postpartum. HMBi was top-dressed onto the total mixed ration of each cow. Treatments did not affect dry matter intake, plasma urea nitrogen, peak milk yield, days to peak milk yield, nonesterified fatty acid, glutamate pyruvate transaminase, glutamic oxalaetic transaminase, milk fat content, milk protein content, milk lactose content, and milk solid non-fat content. The milk composition yields were increased by the HMBi-supplemented treatment. The T1 and T2 treatments increased the yields of 4% fat-corrected milk yield, milk fat, milk protein, and milk lactose compared with the control. Although there was no difference in the milk composition of the control and T2-treated cows, the T2-treated cows exhibited higher milk fat yield (increased by 74 g/d), lower milk urea nitrogen (reduced by 3.41%), and plasma β-hydroxy butyrate than the control cows. The results indicate that HMBi supplementation to diet has beneficial effects, and that there is no difference between supplementation at prepartum and starting only at parturition.
INTRODUCTION
Methionine, one of the most limiting amino acids in dairy rations, is important in dairy cows due to its functions in milk protein synthesis (Guinard and Rulquin, 1995; Pisulewski et al., 1996; Socha et al., 2008). Milk protein synthesis is enhanced when the supply of methionine to the small intestine is increased (Guinard and Rulquin, 1995; Pisulewski et al., 1996; St-Pierre and Sylvester, 2005; Rulquin et al., 2006; Socha et al., 2008). Moreover, increased methionine concentration in metabolizable protein may lead to an increase in milk protein production.
The transition period for dairy cows, ranging from 3 wk prepartum to 3 wk postpartum, is a period marked with significant changes in metabolic demands and endocrine condition (Grummer et al., 1995; Drackley, 1999). Requirements for energy, glucose, and amino acids increase dramatically during this transition period due to parturition and onset of milk production. To partially meet the energy requirements, fatty acids are mobilized rapidly from the adipose tissue, resulting in increased circulating concentrations of nonesterified fatty acid (NEFA) and triglyceride (TG) accumulation in the liver (Bell et al., 1995). Given the potentially detrimental metabolic effects of TG accumulation in the liver during the periparturient period (Bell et al., 1995), methionine is one of the potentially limiting nutrients for hepatic metabolism of fatty acids during the periparturient period. This compound is an essential component of phosphatidylcholine (Bequette et al., 1998), which is important for proper membrane function and in the structure of lipoproteins that transport lipids in the blood. The transport of lipids in the blood may be an important factor in preventing fatty liver disease and ketosis in periparturient dairy cows (Sharma and Erdman, 1988).
However, oral supplementation of methionine might not produce these effects because this amino acid is either quickly degraded to its carbon skeleton and ammonia by the rumen bacteria or incorporated into bacterial protein. Thus, variations on methionine have been developed in an effort to increase postruminal supplies of this compound. Rumen-protected methionine, methionine hydroxyl analogs such as 2-hydroxyl-4-methylthio-butyric acid (HMB) were produced to supply methionine postruminally. The isopropyl ester of HMB (HMBi) has recently been fed to dairy cows. HMBi might be degraded more slowly by the rumen bacteria, providing a steadier supply of methionine to the bacterial populations to affect growth positively (Colleen Fowler, 2009). The effects of amino acid supplementation on growth most likely involve the modulation of gene expression in affected bacterial species (Colleen Fowler, 2009). HMBi is partially hydrolyzed to HMB at the rumen epithelial surface. In the epithelium, HMBi is converted to HMB and apparently exits the epithelium via passive diffusion or possibly at low rates of monocarboxylate transporter-1 (Breves et al., 2010). The passage rates of HMB from HMBi and HMB from HMB through the omasum are 2.3% and 5.3%, respectively (Noftsger et al., 2005). Moreover, HMBi appears to be aminated to methionine through the rumen bacteria and incorporated into bacterial protein. Hence, HMBi supplementation might increase the activities of the rumen bacterial population (Colleen Fowler, 2009). Supplementation of a methionine-deficient diet with HMBi increases milk protein yield and true protein percentage (Rulquin et al., 2006). St-Pierre and Sylvester (2005) also showed that HMBi increases milk, milk fat, and protein yields when fed to cows during early lactation who had been fed a methionine-deficient diet (St-Pierre and Sylvester, 2005). A study compared the production effects of 0.10% HMB, 0.13% HMBi, and 0.088% DL-methionine and found that although both HMBi and methionine increase milk protein content, HMBi causes a higher protein production than methionine (Noftsger et al., 2005).
Therefore, the hepatic fatty acid metabolism, as well as milk protein and milk fat production, is hypothesized to be sensitive to whether HMBi is fed to the dairy cows during the periparturient period. The objective of the present experiment was to compare the effects of feeding HMBi on the performance and health conditions of dairy cows. The experiments were initiated from prepartum d 21 and initiated from the calving day in amounts that resulted in a predicted 3.0:1 ratio of lysine to methionine in metabolizable protein.
MATERIALS AND METHODS
Animals and design
The present experiment was a completely randomized block design arrangement of treatments. Thirty multiparous Holstein dairy cows were blocked based on the expected calving date, previous lactation yield, and body weight. Animals were randomly assigned to three dietary treatments: i) Control, a basal diet; ii) T1, a basal diet plus HMBi (0 g prepartum and 18 g postpartum), and iii) T2, a basal diet plus HMBi (10 g prepartum and 18 g postpartum). HMBi (fed as MetaSmart, Adisseo Life Science (Shanghai) Co., Ltd.) was top-dressed onto the total mixed ration (TMR) of each cow. Treatments were initiated from 21 d before expected calving and continued through 91 d postpartum.
All cows received the same prepartum and postpartum basal diets. Pre- and postpartum basal diets were formulated using the CPM-Dairy (version 3.0.7a) and were predicted to be deficient in methionine and adequate in lysine as described by the National Research Council (NRC, 2001). Additional amounts of HMBi were required to achieve a predicted ration of lysine concentrations in metabolizable protein; the ratio of methionine concentrations in metabolizable protein was 3:1.
Animal management
Cows were housed in free-stall housing from approximately 21 d before expected parturition and fed ad libitum intake with a common prepartum diet thrice daily as TMR. All cows were milked thrice daily, and milk production was recorded at each milking using the Fish Bone Milking Machine (Guangzhou Wanri Milking Systems Co., Ltd). Care and handling of animals were conducted as outlined in the guidelines of the People’s Republic of China National Standard Laboratory Animal Environment and Facilities and the Laboratory Animal Management sections.
Sampling
Milk samples were collected weekly at three consecutive milkings, preserved with 2-bromine-2-nitryl propylene glycol, and refrigerated until analyzed after the third milking. Blood samples were collected via the coccygeal vein on the 7, 21, 35, 60 and 90 d in lactation, for the analysis of blood urea nitrogen (BUN), NEFA, glutamic oxalaetic transaminase (GOT), glutamate-pyruvate transaminase (GPT), and plasma β-hydroxy butyric acid (BHBA) concentration. Blood samples were collected approximately 2 h post-feeding, were immediately centrifuged, and the plasma was removed. Blood plasma was stored for analysis at −20°C.
Sample analyses
Milk samples were analyzed for protein, fat, lactose, and solid non-fat using Ultramilker UL40AC (Hangzhou Ultrasun Technologies Co., Ltd.). Milk urea nitrogen (MUN) were determined through diacetyl monoxime assay using a SkalarSAN Plus segmented flow analyzer (Skalar, Inc., Norcross, GA, USA).
Plasma samples were thawed. GPT, GOT, and BUN concentrations were analyzed by enzymetic colorimetry using the appropriate kit (GPT, GOT, BUN kits supplied by Nanjing Jiancheng Bioengineering Institute). BHBA concentrations were analyzed by enzyme- linked immunosorbent assay with Synergy H4 Hybrid Multi-Mode Microplate Reader (BHBA kit supplied by Wuhan EIAab Science Co., Ltd), whereas plasma NEFA concentrations were determined using a commercial kit (NEFAC Kit, Wako Chemicals, Richmond, VA, USA).
Statistical analyses
Thirty gestating Holstein cows were enrolled in the study. Daily dry matter intake (DMI) and milk yield data were averaged weekly relative to calving prior to statistical analysis.
The milk yield data were analyzed by ANOVA using the SAS MIXED procedure (SAS Institute, 2009). The statistical model was as follows:
where Yijk represents the observations for dependent variables, μ is the overall mean, Bh is the block effect of expected due date and parity, Pi is the effect of time (1–13 wk in lactation), Tj is the effect of treatment, Ck(j) is the random effect of cow within treatment, Pi×Tj is the interaction between time and treatment, and ɛijk is the residual error. The covariance between residuals within cows was modeled as compound symmetry determined by goodness-of-fit measures.
The data for milk composition yield were obtained by the corresponding milk composition content multiplied by the average milk yield of 2 wk before and after sampling dates. These data, as well as the MUN data, were analyzed using the same statistical model as the milk yield.
Blood metabolite and DMI data were analyzed using the SAS MIXED procedure with a repeated measures statement (SAS Institute, 2009). The statistical model for repeated measures includes fixed effects of treatments, time, the interaction of treatment by time, and the first time measured values as well as the random effect of cow within treatment. Time was used in the repeated statement with cow within treatment as the error term and autoregressive covariate structure (Littell et al., 1996).
Overall treatment differences were examined using least-square means with standard error of means (SEM). The Diff and Pdiff options of SAS (SAS Institute, 2009) were used to test treatment differences among least-squares mean. Significance was declared when p≤0.05, and a trend in the data was declared when p≤0.10.
RESULTS
Feed intake and milk production
Results for milk production measurements are reported in Table 2. T1 and T2 treatments increased milk yield by 3.24 and 3.97 kg/d, respectively. The response in milk production to HMBi supplementation was progressive across weeks of lactation (Figure 1).
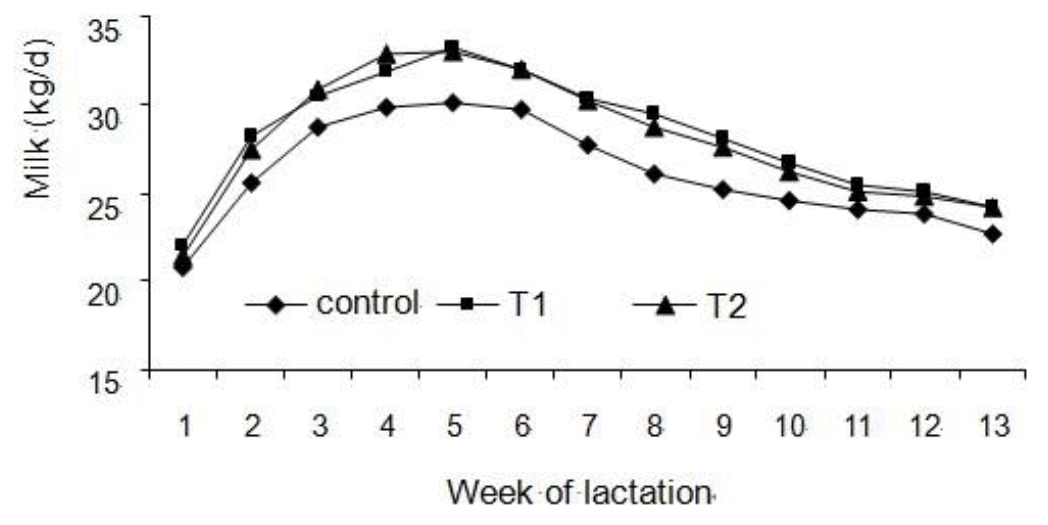
Milk yield of cows from three treatments: Control is basal diet without HMBi; T1 is basal diet plus HMBi initiated from 21 d prepartum to 91 d postpartum; and T2 is basal diet plus HMBi initiated from calving to 91 d postpartum. Milk yield tends to increase for T1- and T2-treated cows compared with the control cows (p = 0.06 and 0.09, respectively).
T1 (3.78%) and T2 treatments (3.89%) increased milk fat content compared with the control treatment (3.69%). Moreover, the milk fat content in T2-treated cows was higher than in T1-treated cows. HMBi supplementation increased milk fat yield compared with cows in the control treatment (1,120 and 1,160 vs. 980 g/d, respectively) (Table 2).
Protein, lactose, and solid non-fat contents of milk were not affected by the treatment (p = 0.90, 0.90, and 0.88, respectively). Milk protein, lactose, and solid non-fat contents averaged 3.55%, 4.92%, and 9.10%, respectively, across the three groups (Table 2).
Supplementation of HMBi increased milk protein yield in T1 and T2 treatments compared with the control treatment (1,050 and 1,040 vs. 950 g/d, respectively) (Table 2). Milk protein yield increased (p<0.01) by 100 g/d−1 in T1, whereas T2 had a significant (p<0.01) increase of 90 g/d (Table 3). T1-treated cows produced an additional 100 g/d of milk protein compared with the control cows (p = 0.01). T2-treated cows tended to have higher milk fat yield than cows in the control (1,087 vs. 1,012 g/d, respectively; p = 0.05).
T1 treatment increased milk lactose and milk solid non-fat yields by 96 g/d (p<0.01) and 248 g/d (p<0.01), respectively. T2 treatment increased milk lactose and milk solid non-fat yields by 94 (p<0.01) and 276 g/d (p<0.01), respectively. There was no significant (p = 0.95) differences in the lactose and solid non-fat yields of T1 and T2. Significant differences for treatment×time interaction for milk lactose (p<0.01) and milk solid non-fat (p<0.01) yield demonstrate that T1- and T2- treated cows responded significantly by increasing milk lactose and milk solid non-fat yields in the first 13 wk of lactation.
T2 treatment tended (p = 0.07) to decrease MUN compared with the control treatment, whereas the T1 treatment did not affect MUN (p = 0.66). MUN concentration tended to be reduced by 3.41% in T2-treated cows compared with those in the control.
Peak milk yield (p = 0.62) and days to peak milk yield (p = 0.46) did not differ among treatments, which averaged 34.10 kg/d (SEM = 0.73) and 30.8 d (SEM = 1.3) across the three treatments.
Plasma metabolites
Least-square means of plasma metabolite concentrations are presented in Table 3. Treatments did not affect plasma GOT, GPT, NEFA, and BUN concentrations. T2-treated cows had lower BHBA concentration compared with those in the control treatment (4.98 vs. 5.21 mg/dl, p<0.01). T1- and T2-treated cows had no significant differences (p = 0.95 and 0.98, respectively) in lactose and BHBA concentrations (p = 0.68).
DISCUSSION
Dry matter intake
Ordway (2009) reported that the prepartum DMI does not vary when cows are fed approximately 0.35% and 0.54% HMBi in diet dry matter during prepartum and postpartum periods, respectively. However, when DMI was analyzed by week for all cows, postpartum differences in DMI are significant during wk 4 to 5 for cows fed with 0.54% HMBi in diet dry matter during the postpartum period. When DMI was analyzed by parity, primiparous cows receiving HMBi show higher DMI during wk 4 to 7 and wk 15 postpartum than the cows receiving the control diet (Ordway et al., 2009). In our present experiment, T2-treated cows consumed an average of 10 g/d HMBi during the prepartum period and 18 g/d HMBi during the postpartum period, whereas T1-treated cows consumed 0 g/d HMBi during the prepartum period and 18 g/d HMBi during the postpartum period. No significant effects of HMBi on DMI was observed, which is in agreement with the results of previous experiments with HMBi (St-Pierre and Sylvester, 2005; Rulquin et al., 2006; Phipps et al., 2008).
Milk yield and composition
Research has indicated that methionine is often a limiting amino acid for ruminants fed with corn silage- or haylage-based diets. Several studies have shown that supplementing diets with HMBi increase lactation performance. In the present study, HMBi supplementation of the diets improved milk yield and composition, in contrast to the results reported by Rulquin (2006) and Phipps et al. (2008), but is consistent with those of St-Pierre and Sylvester (2005). Feeding the cows with HMBi increases milk protein yield compared with the control diet (Noftsger et al., 2005; St-Pierre and Sylvester 2005; Rulquin et al., 2006; Socha et al., 2008). We also observed increases in the T2 treatment and a trend toward an increase in milk fat yield in T1 treatment, which is not consistent with the results of the previous experiment. The T2 treatment tended (p = 0.07) to reduce MUN concentration by 3.41% compared with the control, in contrast with the results reported by St-Pierre and Sylvester (2005). The milk yield and composition response to HMBi supplementation are inconsistent with the literature. The Latin square design (short-term period) apparently did not allow HMBi to produce significant effects on the milk yield because the results of the study by St-Pierre and Sylvester (2005) and our work indicate that a prolonged period would mediate response to HMBi on milk yield.
A similar study by Ordway et al. (2009) showed no difference in the milk yield, milk fat yield, milk true protein yield, milk lactose yield, and MUN concentration. However, in the present study, supplementation with HMBi enhanced milk protein concentration compared with the control treatment (2.81% vs. 2.71%). This discrepancy is not fully understood because the experimental conditions (lactation stage, energy, offered form, and HMBi source) were very similar to those of Ordway et al. (2009). However, compared with the aforementioned study, the amount of HMBi fed (approximately 10 vs. 47.25 g/d prepartum, 18 vs. 123 g/d postpartum, respectively) and the basic diets were different in our experiment. Furthermore, the difference between the milking levels for experimental cows (with an average of 27.43 kg/d in our study vs. 43.0 kg/d in the study of Ordway et al., 2009) would affect the response to HMBi for lactation performance. Thus, future research should be conducted to determine the effect of different HMBi-supplemented levels on milking performance for varied lactating production level cows.
In the current study, there was no difference between the milk yield and milk composition yield for the supplementation beginning at prepartum and that beginning only at parturition. The results indicate that the short period within which supplementation beginning at prepartum HMBi was given does not allow HMBi to produce significant effects on milk yield.
Plasma metabolites
In the current study, no difference in plasma GPT, GOT, NEFA, and BUN concentrations was noted for all treatments. However, T2 treatment reduced plasma BHBA concentration in the cows, which disagrees with the absence of effect as observed by Rulquin (2006). Plasma metabolite concentrations are affected by numerous other factors. Thus, further studies must be conducted to determine whether HMBi supplement affects plasma metabolite concentration.
CONCLUSION
Supplementation of HMBi beginning at prepartum and starting only at parturition increases milk, protein, milk lactose, and milk solid non-fat yields. However, in the present study, with the exception of lower MUN and plasma BHBA concentrations when HMBi supplementation was initiated from prepartum, we did not find any difference between the results of supplementation beginning prepartum and beginning only at parturition. Therefore, the beneficial effects of HMBi supplementation to diet and the effects of prepartum supplementation are similar to the effects of postpartum supplemented HMBi.
ACKNOWLEDGEMENTS
This work was financially supported by the National Dairy Industry Research System (nycytx-02-02) and the Public Industry (Agriculture) Research Special Funds of China (No. nyhyzx07-036-06).